Metal Absorbing Laser Mechanism
The reflection, absorption and transmission of light on the surface of the material are essentially the result of the interaction between the electromagnetic field of light waves and the charged particles of the material. Metals have a high density of free electrons, which are forced to vibrate by the electromagnetic field of light waves, producing secondary electromagnetic waves (subwaves).
Interference between these subwaves and between the subwaves and incident waves results in strong reflected waves and relatively weak transmitted waves, which are absorbed by a thin metallic surface layer. Therefore, the metal surface generally has a high reflection rate in relation to the laser. Especially for low-frequency infrared light, its photon energy is low, mainly affecting the metal's free electrons, reflecting strongly.
For high-frequency visible light and ultraviolet light with higher photon energy, they can affect the electrons bound to the metal. The action of the bound electrons will reduce the metal's reflectivity, increase its transmissibility, and increase the metal's laser absorption.
Due to the high density of free electrons, the transmitted wave is absorbed by a very thin surface layer of metal. Measurements from ultraviolet light with a wavelength of 0.25 μm to infrared light with a wavelength of 10.6 μm show that the penetration depth of light into various metals is only 0.01 ~ 0.1 μm.
As explained above, the penetration depth is equal to the inverse of the linear absorption coefficient, therefore the linear absorption coefficient of metal to light waves is large, between 10 5 ~10 6 cm -1 .
After absorbing the laser, the material converts light energy into thermal energy, exciting resonance of charged particles and collisions between particles. The entire process is completed in a very short time. The total energy relaxation time for metals is typically 10 -13 S. For general laser processing, it is believed that the conversion of absorbed laser to thermal energy is completed instantaneously.
At this moment, the heat is confined to the laser irradiation area of the material. Subsequent heat conduction transfers heat from high temperature areas to lower temperature areas.
Factors Affecting Laser Metal Absorption
Laser absorption by metal is related to a series of factors such as laser wavelength, material properties, temperature, surface condition and polarization characteristics.
(1) Influence of laser wavelength
Generally speaking, the longer the laser wavelength, the higher the reflection rate and the lower the absorption rate.
Figure 1-2 shows the relationship between the reflection ratio R of commonly used metals at room temperature and wavelength. In the infrared region, the absorption rate A is approximately proportional to (ρ/λ) 1/2 , where ρ is the resistivity of the material and λ is the wavelength. As the wavelength increases, the absorption rate A decreases and the reflection rate R increases.
The absorption rate A of various metals at different laser wavelengths at 20°C is shown in Table 1-1.
As can be seen from Table 1-1, at room temperature, the absorption rate of the 10.6 μm wavelength infrared laser on the metal surface is almost an order of magnitude lower than that of visible light (the wavelengths laser wavelength in the table are 500nm and 700nm), and the absorption rate of the 1.06μm YAG infrared laser is significantly higher than that of the CO 2 laser.
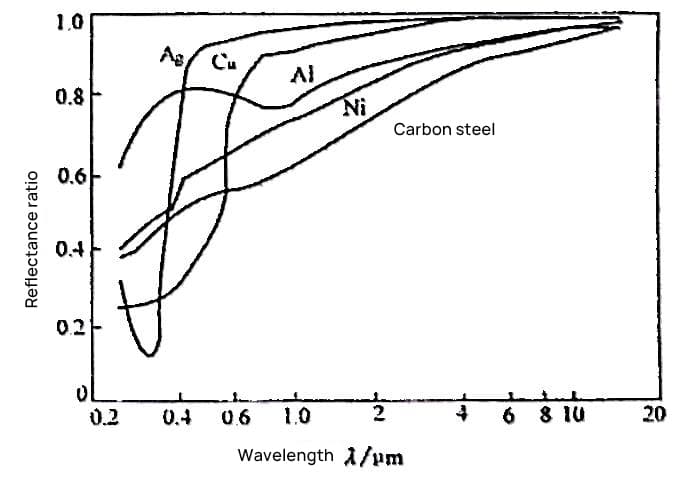
Table 1-1: Absorption rates of various metals at different laser wavelengths at 20°C
Materials | Argon Ion | Ruby | YAG | CO2 |
Wave-length | 500nm | 700nm | 1.06μm | 10.6μm |
Aluminum | 0.09 | 0.11 | 0.08 | 0.019 |
Copper | 0.56 | 0.17 | 0.1 | 0.015 |
Gold | 0.58 | 0.07 | – | 0.017 |
Iridium | 0.36 | 0.3 | 0.22 | – |
Iron | 0.68 | 0.64 | – | 0.035 |
Lead | 0.38 | 0.35 | 0.16 | 0.045 |
Molybdenum | 0.48 | 0.48 | 0.4 | 0.027 |
Nickel | 0.4 | 0.32 | 0.26 | 0.03 |
Niobium | 0.58 | 0.5 | 0.32 | 0.036 |
Platinum | 0.21 | 0.15 | 0.11 | 0.036 |
Nickel | 0.47 | 0.44 | 0.28 | – |
Silver | 0.05 | 0.04 | 0.04 | 0.014 |
Tantalum | 0.65 | 0.5 | 0.18 | 0.044 |
Tin | 0.2 | 0.18 | 0.19 | 0.034 |
Titanium | 0.48 | 0.45 | 0.42 | 0.08 |
Tungsten | 0.55 | 0.5 | 0.41 | 0.026 |
Zinc | – | – | 0.16 | 0.027 |
(2) Impact of material properties
As shown in Figure 1-2, in the visible light region and adjacent areas, different metals exhibit complex variations in reflectance. However, in the infrared range where λ>2μm, the order of reflectance for metals is: Silver > Copper > Aluminum > Nickel > Carbon Steel, which indicates that the better the conductivity of the material, the greater its reflectance to infrared radiation.
This rule can be explained by the mechanism of laser absorption by these metals: in this infrared range, the photon energy is low and can only couple to the metal's free electrons. The lower the resistivity of the metal, the greater the density of free electrons. The forced vibration of free electrons produces stronger reflected waves, resulting in a higher reflectance rate.
Calculations show that there is an approximate relationship between the absorption rate and the resistivity of the metal as follows:

In the formula:
A represents the absorption rate of the metal by the laser;
ρ is the resistivity of the metallic material at the time of measurement (Ω·cm);
λ is the laser wavelength (cm).
This relationship was confirmed by tests on different polished metal surfaces.
(3) The impact of temperature
The resistivity of the metal increases with increasing temperature, which shows that:

In the equation:
ρ 20 is the resistivity of the metallic material at 20°C (Ω·cm);
γ is the temperature coefficient of resistance (℃-1);
T is the temperature (℃).
By substituting equation (1-14) into equation (1-13), we can calculate the absorption rate at different temperatures, which is given by:

As can be seen from equation (1-15), the absorption rate increases with increasing temperature. This relationship is not only applicable to solid metals, but is also valid for liquid metals.
Table 1-2 lists the resistivity p20 and the temperature coefficient of resistance γ for various metals at 20°C. Figure 1-3 shows the change in the absorption rate of various metals for an infrared laser of wavelength 10.6 μm with temperature, calculated according to equation (1-15).
It is observed that although the temperature coefficient of resistance for low carbon steel is not significantly different compared to aluminum and copper, its resistivity at 20°C is much higher, making its absorption rate not only higher in absolute value, but also increases more rapidly with temperature. . However, in general, the polished surfaces of most solid metallic materials have a low absorption rate relative to a 10.6 μm wavelength laser, none greater than 11%.
Table 1-2: Resistivity ρ 20 and temperature coefficient of resistance γ for various metals at 20 ℃.
Materials | ρ 20 (Ω·cm) | γ(℃ -1 ) | Materials | ρ 20 (Ω·cm) | γ(℃ -1 ) |
Aluminum | 2. 82×10 -6 | 3. 6×10 -3 | Nickel | 7.24 × 10 -6 | P. 4×10 -3 |
Brass | 8.00×10 -6 | 1.5×10 -3 | Platinum | 1.05×10 -5 | 3. 7×10 -3 |
Bronze | 8h00×10 -6 | 3. 5×10 -3 | Silver | 1.62×10 -6 | 3.6 × 10 -3 |
Copper-nickel alloy | 4. 90×10 -5 | 1.0×10 -5 | steel alloy | 1.50×10 -5 | 1.5×10 -3 |
Copper | 1.72×10 -5 | 4. 0×10 -3 | Low carbon steel | 1.50×10 -5 | 3.3×10 -3 |
Gold | 2. 42×10 -6 | 3. 6×10 -3 | Structural Steel | 1. 20 × 10 -5 | 3. 2×10 -3 |
Invar | 7. 80×10 -5 | 2. 0×10 -3 | Tantalum | 1.55×10 -5 | 3.1×10 -3 |
Iron | 9.80×10 -6 | 5. 0×10 -3 | Tin | 1. 14 × 10 -6 | 4. 0×10 -3 |
Manganese | 4. 40×10 -6 | 1.0×10 -5 | Cadmium | 5.50×10 -5 | 5.2×10 -3 |
Molybdenum | 5. 60×10 -6 | 4. 7×10 -3 | Zinc | 5. 92×10 -5 | 3.5×10 -3 |
Nichrome Alloy | 1. 00 × 10 -4 | 4. 0×10 -4 |
(4) Impact of surface condition
The roughness of the metal surface, the state of the oxide film and the presence of special surface coatings can significantly affect the absorption rate of infrared lasers.
The absorption rates in Table 1-1 were measured using smooth metal surfaces in a vacuum. However, real laser-heated metal surfaces, due to oxidation and contamination, have much higher absorption rates for infrared lasers than the values in the table. The impact of surface condition on the absorption rate of visible light is relatively small.
Table 1-3 shows the effect of the surface condition of aluminum and its alloys on the absorption rate of CO 2 lasers.
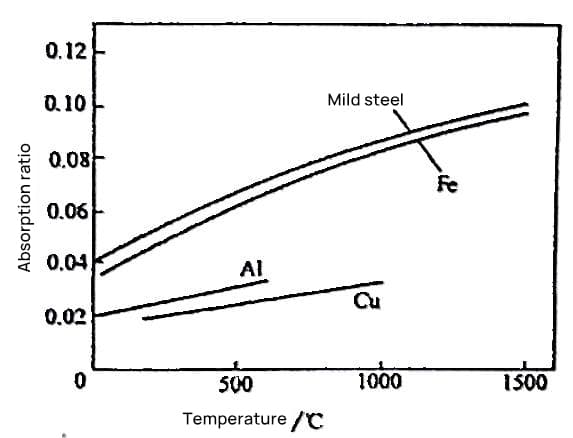
Table 1-3: Impact of the surface condition of aluminum and its alloys on the absorption rate of CO 2 laser (6) (%).
Materials | Original surface | Electropolishing | Sand blasting | Anodizing |
Pure Aluminum | 7 | 5 | 20 | 22 |
5456 Aluminum Alloy | 5~11 | 4 | 22 | 27 |
The oxide film formed on metal materials at high temperatures significantly increases the absorption rate. Figure 1-4 shows the relationship between the absorption rate of an infrared laser of wavelength 10.6 μm on the surface of 304 stainless steel oxidized in air for 1 minute and the oxidation temperature; Figure 1-5 shows the relationship between the absorption rate of a molybdenum surface and the same laser with the oxidation temperature and time.
Since the thickness of the oxide film is a function of oxidation temperature and time, the laser absorption rate is also affected by oxidation temperature and time. The absorption rate of metallic materials for a wavelength of 10.6 μm CO 2 Laser increases significantly with temperature, due to two factors: increasing resistivity and surface oxidation at high temperature.
Phosphates, zirconia, titanium oxide, silica, as well as carbon black, graphite, etc., are substances with high absorption rates for CO 2 lasers. Surface coatings mainly composed of these substances can significantly increase the absorption rate of metals relative to infrared lasers, which has become an important measure that must be taken during laser surface heat treatment.
However, for laser welding, the increase in phosphorus, oxygen and carbon is very harmful. They can reduce the plasticity and toughness of the weld seam and their use should be carefully considered.
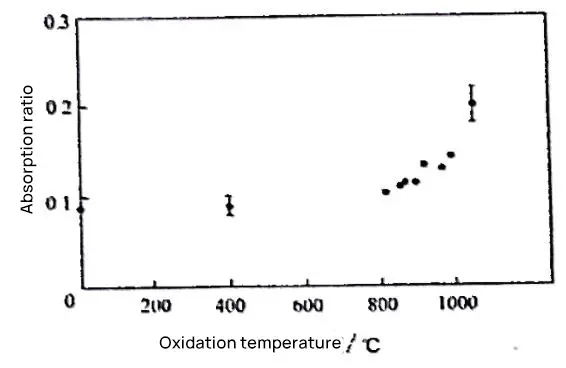
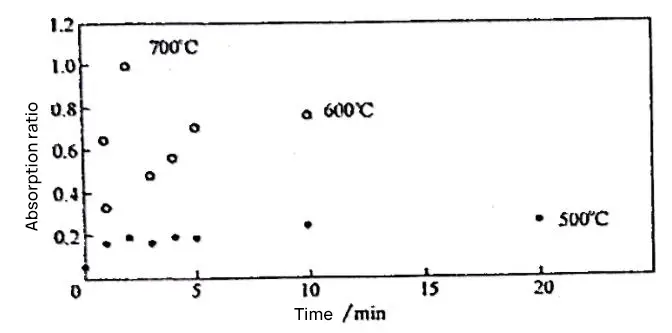
(5) The Impact of Polarization
Since the incident laser is not perpendicular to the material surface, the reflection rate and absorption rate are related to the polarization state of the incident laser. This issue was discussed in section 1.1.1.