1 . Preface
Compared to traditional welding methods, laser welding (as illustrated in Figure 1) offers several advantages, including:
- Centralized and adjustable power density
- No physical contact with the part to be welded
- High welding efficiency
- A tight, strong weld seam
As a result, laser welding is widely used in equipment manufacturing industries such as automobiles, ships, and aerospace, and is expanding into new material processing applications.
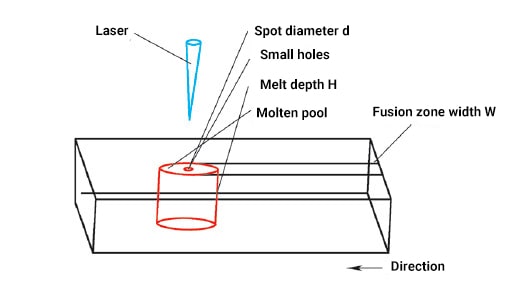
Figure 1 Principle of laser welding
To remain competitive in the global industrial market, major industrial countries have introduced strategies aimed at modernizing and upgrading their industries. Examples of these initiatives include Germany's Industry 4.0 and the American Industrial Internet, which aim to promote technological innovation and provide critical financing for the industrial sector.
Laser welding, as a crucial component of advanced equipment technology, has received significant attention in these efforts. To meet welding needs, several new laser welding technologies have been proposed to solve practical problems.
For example, Professor W. Steen of Imperial College London introduced the concept of laser-arc hybrid welding. This technology addresses the limitations of traditional laser welding and expands its range of applications. The combination of laser and arc welding enhances the advantages of both, reduces welding gap size requirements, minimizes cracks and pores during welding, and improves welding component performance.
So far, laser welding technology has developed into several types, such as:
- Heat conduction laser welding
- Laser Deep Penetration Welding
- Laser Wire Filler Welding
- Laser-arc hybrid welding
- Remote laser scanning welding
- Laser brazing
Intermediate process control technologies such as laser seam tracking and real-time monitoring of the welding seam process with high-speed cameras have been developed to solve the limitations and shortcomings of laser welding. Furthermore, defect processing has also been implemented to further improve the laser welding process.
2. Progress of research at home and abroad
In recent years, domestic and foreign research teams have been actively exploring and studying the most suitable process parameters for laser welding from the perspective of laser movement and heat source combination. This has led to improvements in several laser welding methods, including deep penetration laser welding and laser-arc hybrid welding.
Research on laser welding is not limited to appearance alone, as modern characterization methods such as high-speed cameras and spectral analysis are being used to study the characteristics of the welding process and understand the mechanism of weld defect formation.
However, the internal changes during laser welding are complex. To address this, research teams have experimented with applying external energy sources, such as magnetic fields, multiarcs, and electric fields, to the laser welding process. The aim is to reduce weld defects, improve mechanical properties and improve overall welding quality.
2.1 Research on laser welding technology
Laser welding can provide high-quality joint strength and deep welding with a large depth ratio. Compared with traditional welding methods, it features higher power density and better welding effect on difficult-to-weld materials, as well as the ability to weld materials of varying properties. This motivated extensive research by both national and international scholars.
In China, the focus of laser technology research mainly centers on the parameters of each welding process, such as welding speed, laser power, defocus amount, laser pulse waveform and shielding gas flow. Researchers also study the mechanical properties, structure evolution and regulation of welded joints.
Laser pressure welding is a unique form of laser welding that combines laser-induced heating with traditional flat seam welding. The process involves partially melting the part with a laser beam, followed by rolling under high pressure to produce the welded joint. This technology is advantageous because it avoids welding defects such as shrinkage and gas cavities due to the narrow fusion zone. It is also suitable for connecting thin boards.
The research team conducted a study on the evolution of the structure during laser pressure welding of pure aluminum, as illustrated in Figure 2. The team investigated the basic aspects of the evolution of the microstructure during the welding process of pure aluminum. Through a thorough analysis of the microstructure of the sample during the laser pressure welding process, it was concluded that the solidification process began before lamination, causing the newly crystallized material to undergo plastic deformation.
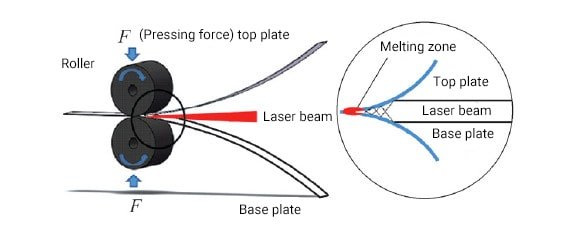
Figure 2 Main diagram of laser pressure welding
Hybrid laser-arc welding, as illustrated in Figure 3, is a promising processing method of the 21st century and has been extensively researched by scholars. The researcher studied the welding of 50CrV/SPHE dissimilar steel by adjusting the process parameters and analyzed the impact on weld formation and droplet transfer.
Research results indicate that the ideal laser power range is between 2800 and 3400 W, which leads to uniform heating of the welding wire and a stable welding process. By combining oscillating scanning with laser-arc hybrid welding, weld defects can be corrected.
The research team also welded aluminum alloy materials using horizontal, vertical and circular oscillation scanning methods. High-speed cameras and spectroscopy were used to analyze changes in the droplets. The results showed that the optimized parameter range for the circular scanning method is greater than that of the horizontal and vertical directions, promoting interaction with the plasma to form droplets with smaller diameters, which is beneficial for grain refinement.
In comparison, plasma arc energy is more concentrated, and laser-plasma arc welding is found to have good adaptability to gaps and misaligned edges in flat welding.
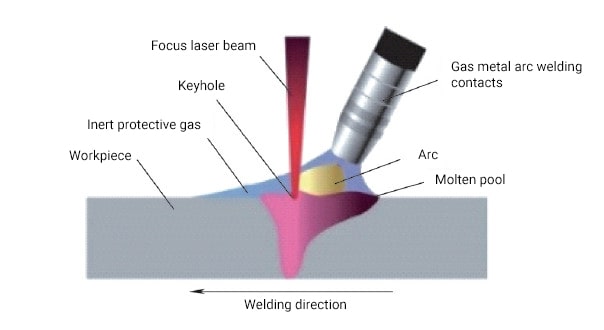
Figure 3 Hybrid arc-laser welding
International research into welding technology has focused on improving welding conditions and incorporating external energy. In order to explore the potential of this process to connect large, safety-critical nuclear components, such as steam generators or pressurized water reactor (PWR) boosters, vacuum laser welding technology was employed. The technology was used at a speed of 150 mm/min with a 16 kW laser to produce 80 mm thick SA5083 steel welds in two welding passes.
The advantages of vacuum laser welding were presented and compared to electron beam welding in terms of the physics of the process. It was concluded that vacuum laser welding deserves further development due to its potential to support future nuclear power construction plans.
Bunaziv I et al. investigated cold metal transfer pulse arc (CMT+P) mode when using fiber laser-MAG hybrid welding. The team used metallic wire to weld 45 mm thick high-strength steel (double butt-to-side welding) and compared the influence of different pulses and the front and rear pilot arc on the weld.
When compared to traditional pulsed arc welding, it has been found that both methods can provide high quality welding. However, CMT+P mode provides more stable droplet transfer within a limited range of feed speed.
2.2 Laser welding process control
Laser welding technology is a non-contact welding method that features faster speed and higher efficiency. Intermediate process treatment plays a critical role in determining the quality of the welded joint.
In China, laser welding process control (as shown in Figure 4) mainly focuses on monitoring the welding process with the aid of optical devices. For example, laser welding seam tracking and high-speed cameras are used to monitor the weld seam in real time.
An example of this monitoring is the use of a high-speed camera system to observe in real time the process of formation of pores and spatter during laser welding of high-strength galvanized steel DP780. The pore escape route was studied from a dynamic perspective.
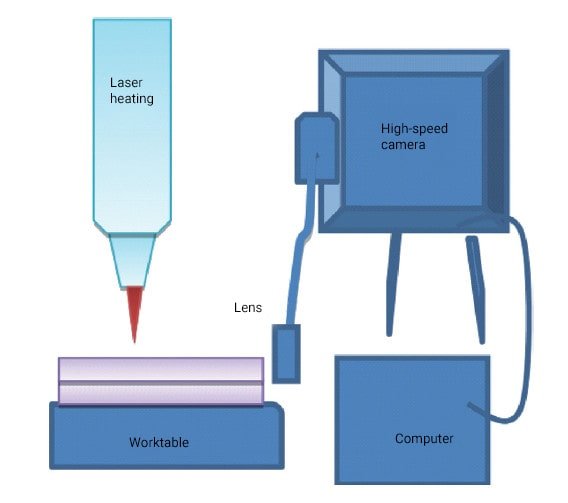
Figure 4 Layout of the welding test process
The laser welding head is equipped with a CCD video tracking module, and an automatic weld bead detection method using a line laser has been proposed. This method takes advantage of laser triangulation to collect information such as weld height and width.
The principle of straight-line laser detection is demonstrated in Figure 5. During laser welding, a straight-line laser beam is directed vertically toward the welding seam, and the image is captured on the CCD image plane through reflection diffuse from the upper surface of the workpiece. Each weld characteristic point on the image plane corresponds uniquely to a point on the part surface.
For tracking algorithms, a high-precision and fast-speed nuclear filter target tracking algorithm is used to track the positions of straight and curved welds respectively. The error between the data fitting curve and the experimentally obtained weld shape is within 5%, demonstrating high agreement and providing a good real-time tracking effect.
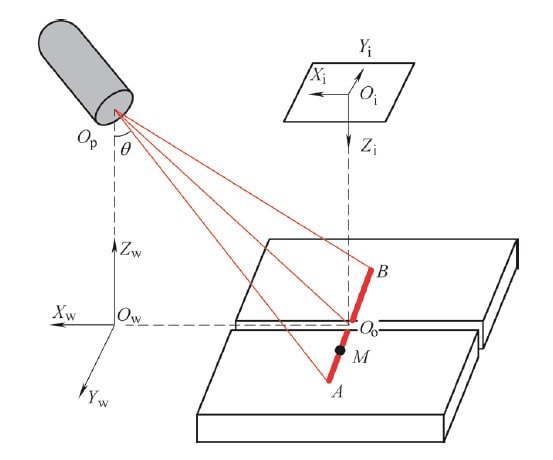
Figure 5 Principle of straight line laser detection
Foreign research has focused on investigating the integration of external energy in the welding process and the use of artificial intelligence to simulate and predict welding results. The study of welding copper materials used in the interconnection of lithium-ion batteries and high-power electronic devices was carried out by combining additional parameters such as frequency and amplitude of oscillation with the spatial power modulation method that involved a linear supply with circular motion overlaid. The results indicate that not only the size of the connection area can be increased, but also the stability and quality of the laser welding process.
However, when welding certain specialty metals, the weld may not fully mix into the weld pool, leading to uneven distribution of elements in the weld. To solve this problem, the researchers used an oscillating magnetic field to generate a non-conservative Lorentz force component in the molten pool, thereby improving the distribution of elements throughout the thickness of the material. Spectroscopy (EDS) was used to analyze the distribution of two trace elements (Ni, Cr), and the results showed that rotating the magnetic field 30° in the welding direction significantly improved the weld distribution.
This research provides strong evidence for the effectiveness of magnetic fields in welding. Belitzki proposed a method to minimize deformation in complex structures with multiple welds, using an artificial neural network to establish a metamodel that predicts local deformation based on welding parameters in subareas. The genetic algorithm was employed to find the optimal welding parameters that would result in the lowest deformation overall. The results indicate that this method can effectively and accurately identify the best parameters among more than 1 billion potential combinations.
2.3 Laser welding defect treatment
The application of laser welding is widespread, but the process is often accompanied by defects such as cracks, pores and spatter.
Considerable research has been conducted both nationally and internationally. The researchers employed oscillation, pulse and other techniques in combination with laser welding.
Studying the principle of laser welding gives equal importance to integration with industrial equipment and the use of new products to boost research efforts. Research is highly practical.
National research mainly focuses on finding solutions for defects in laser welding joints and investigating the formation mechanisms of these defects in detail. The teams use simulation analysis, scanning electron microscopy and other methods to study issues such as melt pool splash and Fresnel absorption effect.
When high-powered lasers are aimed at the work surface, they quickly vaporize the material and create a keyhole. The Fresnel absorption effect of the molten pool and keyhole determines the welding quality.
Figure 6 illustrates porosity defects caused by laser welding of DP780 high-strength galvanized steel. Research into the keyhole and Fresnel absorption of laser deep penetration welding has revealed that multiple laser reflections within the keyhole result in an unequal total power density of Fresnel absorption, with greatest density near the bottom of the keyhole. keyhole than at the top. Laser reflection is a crucial factor affecting this density distribution.
Single focus laser welding has certain limitations. For example, temperature control during welding is not possible and the high thermal sensitivity of the material can lead to the formation of cracks in the weld.
To stabilize the welding process, many researchers have studied dual-focus laser welding. Some have examined keyhole stability and molten pool flow of aluminum alloys in a dual-focus laser array.
A coupling model was established for the welding transient molten pool and internal flow in dual-focus laser welding of aluminum alloys, using the ray-tracing method to create a heat source model that takes into account the absorption effect. Fresnel, steam recoil force and internal flow of the weld pool.
Research results indicate that dual-focus laser welding is more stable and controllable, with significantly weaker keyhole fluctuation compared to single laser welding.
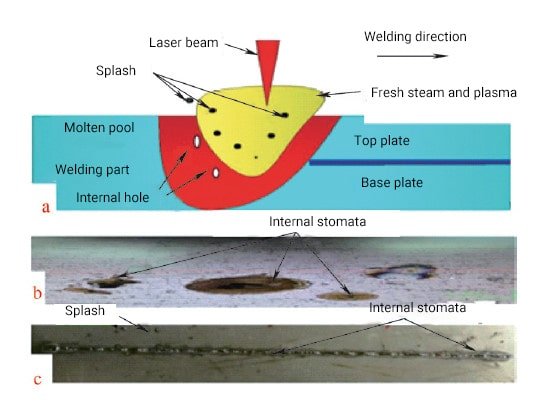
Figure 6 The principle of pore defects in laser deep penetration welding
Compared with foreign countries, domestic research mainly focuses on changing the morphology of the laser beam, with most studies devoted to investigating the impact of the number of laser beams on laser welding defects.
Foreign research groups have made efforts to discover the formation mechanism of keyhole collapse and molten pool splash through the use of new optical components.
Additionally, some foreign researchers have attempted to improve laser welding by introducing new techniques such as beam oscillation or laser power modulation in an effort to reduce defects.
Volpp J. employed a newly developed multifocal beam shaping optical element, which can generate multiple laser beams in the axial direction. This component can be used to alter the energy input into the keyhole and surrounding areas, providing insight into the spatter formation mechanism and evaluating the potential of axial beam shaping to reduce defects in deep penetration laser welding.
The results reveal that under exposure to high-intensity light, the number of splashes can be effectively reduced, keyhole collapse is prevented, the upper section of the keyhole receives sufficient energy input, and liquid splashes are minimized.
3. Laser welding application status
After several years of research and development, laser welding technology has been successfully implemented in various equipment manufacturing industries, including the automobile, oil and gas pipeline, and tram equipment industries.
This article will focus on introducing the main components of laser welding system and their practical applications in material processing engineering.
3.1 The main components of the laser welding system
(1) generator laser
The laser generator is the central component of the laser welding system and is responsible for producing laser light.
Lasers are composed of three essential parts: an excitation system, a laser active medium, and an optical resonant cavity.
Over the years, the performance of lasers has advanced greatly and there are several types of lasers available, including fiber lasers, semiconductor lasers, CO2 lasers, and others, as illustrated in Figure 7.
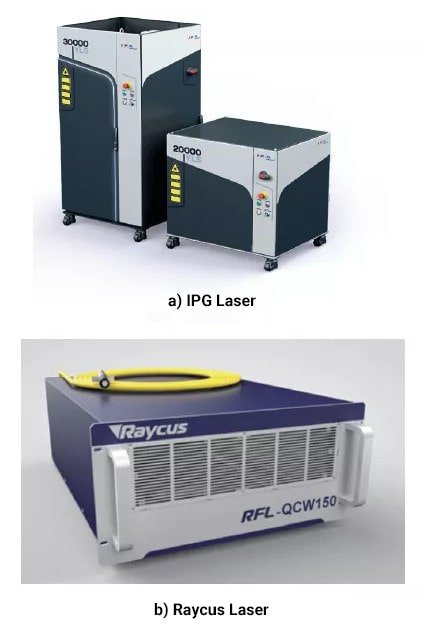
Figure 7 Laser generator
Foreign laser companies of exceptional quality include Coherent and Trumpf, which have inherent advantages in their laser technology. After extensive research and development, its lasers have achieved high beam quality, high photoelectric conversion efficiency and exceptional stability.
The spot produced by a semiconductor laser is more focused compared to that of a fiber laser, with more uniform energy distribution and lower power consumption. For example, the TruDiode series of high-efficiency semiconductor lasers has won users' trust with its excellent results, low investment costs and operating expenses. This laser can provide stable laser power of up to several kilowatts.
Some typical applications for these lasers include deep penetration welding, heat conduction welding, laser metal cladding, brazing and plastic welding, with an efficiency of up to 40% that reduces production operating costs. The TruDiode laser is also highly sophisticated as it does not require extra resonant cavity structure.
CO2 lasers are a popular type of gas laser that can utilize the energy level structure of CO2 molecules to produce spectral output in multiple wavelength bands. They have superior thermal performance compared to solid-state lasers and can store a large amount of heat, making them ideal for high-power laser applications.
The national laser industry has the advantage of being able to update quickly. After years of technical research, numerous prominent laser companies have emerged in China, such as Raycus Laser and Chuangxin Laser. With their excellent laser products, competitive prices and localized product strategies, these companies have captured a significant share of the domestic laser market.
Figure 7b shows the near-continuous fiber laser produced by Raycus. This laser has a small power ranging from 75 to 300W, with improved compatibility, higher electro-optical conversion efficiency, better beam quality and lower maintenance costs. It is ideal for industrial applications that require long pulse widths and high peak values, such as laser spot welding and laser seam welding.
(2) Laser welding head
With the advancement of laser welding technology, various types of laser welding heads have been introduced to meet diverse welding needs and functions. Figure 8 shows these different types of laser welding heads.
Starting from the left is the welding head, followed by the laser galvanometer scanning head, and finally the dual spot welding oscillating head and beam shaping head. The latter can support power of up to 50kW.
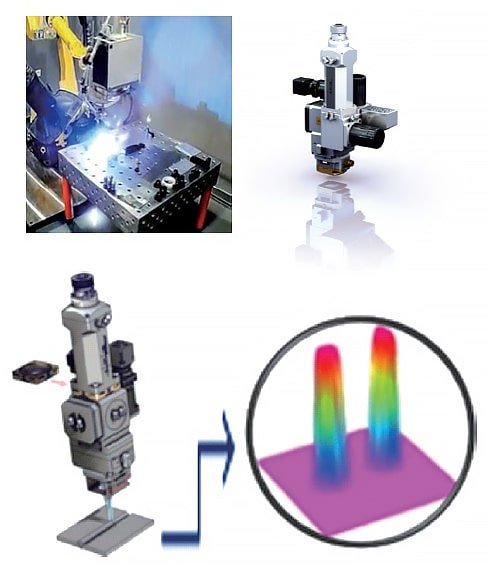
Figure 8 Common Laser Welding Head
The design and application of the welding head are based on actual welding requirements, providing solutions for different welding needs. For example, when the laser needs to be divided into multiple beams to increase welding efficiency, using a scanning galvanometer welding head can effectively meet the requirement of high efficiency.
As shown in Figure 8, the oscillating welding joint can significantly improve the internal and external weld quality, as well as improve the weldability of defect-prone materials.
3.2 Engineering application of laser welding technology
Laser welding has been used in the automotive industry and other areas since its inception, as shown in Figure 9. Over time, its use has expanded to the shipbuilding, aerospace, semiconductor, electronics and consumer products industries. It has moved from traditional fields to more diverse and in-depth material processing applications.
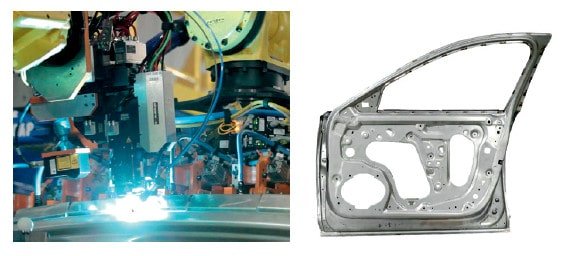
Figure 9 Laser welding applications in the automotive sector
The automobile manufacturing process mainly employs laser welding technology for custom welding of body plates with varying thicknesses, body welding and welding of automotive parts.
The use of laser welding technology reduces the weight of the car body, leading to greater energy efficiency and reduced emissions. It also reduces stamping and assembly costs during the manufacturing process, increases assembly accuracy, rigidity and overall car body integration, thereby improving comfort and safety.
Laser welding has become a popular method in the automotive industry. Figure 9b illustrates the workshop of a national auto parts company, where the car door is laser brazed and welded. The company uses a larger laser spot of 2 to 4 mm and a laser power of 2 to 4 kW and employs contact tracking to test edge nodes. After calibration, it was found that the weld beads were narrower than those produced by other welding methods, resulting in a better overall appearance of the car body. Tests showed that the strength of the welds was significantly improved compared to traditional welding.
When using laser welding, it is important to select the appropriate shielding gas according to the material to be joined. Laser welding is faster, more efficient, requires a smaller work area and produces less deformation than other methods. In some cases, it eliminates the need for heat treatment to reduce residual stress.
Laser welding technology can greatly improve the quality of welded products and increase efficiency in the manufacturing industry, including medical devices. No adhesives are required during the welding process and very little slag or welding debris is produced. This has greatly contributed to the development of the medical device industry.
Plates used in shipbuilding differ from those used in other mechanical products, and laser welding technology can effectively solve problems with longer weld beads and warping in ship plates. The laser welding process has a wide range of engineering applications, including welding seam positioning, cross-sectional scanning and online monitoring of surface formation.
Figure 10 shows the new LDD-700 laser welding whole process monitoring system, which is based on coherent interference imaging technology. Its 3D imaging mode allows the LDD-700 to adapt to changes in keyhole geometry from different processes, providing the basic capability for accurate depth measurement. The system's powerful software supports customized monitoring solutions to meet the diverse requirements of different processes.
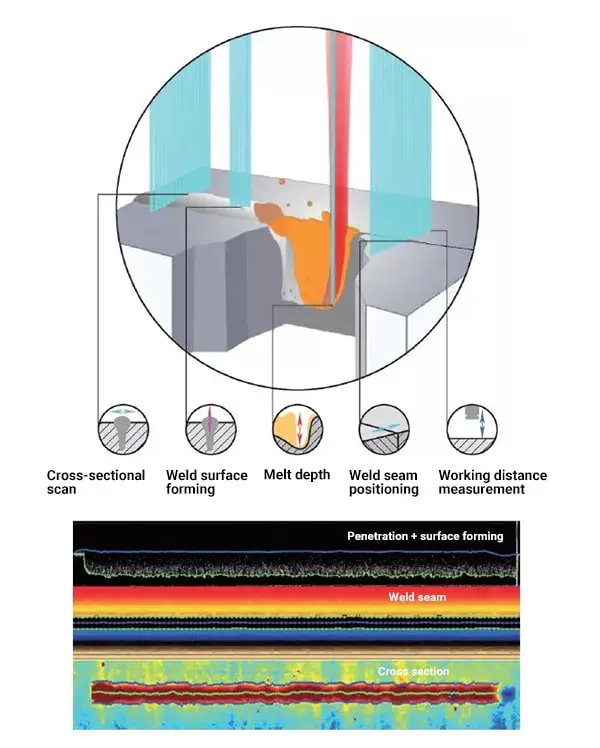
Figure 10 Engineering application of laser welding monitoring process
Laser welding is also widely used in connecting oil pipelines. The implementation of robotic laser welding improves the efficiency and reliability of the welding process, as well as the quality of welded joints.
As a cutting-edge high-energy beam welding technology, laser welding has several advantages, such as no need for a vacuum environment, focused heat input, minimal thermal deformation, high weld aspect ratio, high precision and the ease of achieving automatic welding.
It has been determined that laser welding is the most suitable method for sealing the DC coil case.
4. Summary and perspectives
The development of laser welding has made great progress through engineering research and application in process processing, welding process treatment and welding defect resolution.
Research in this area focuses mainly on two aspects: improving the laser welding process and exploring new techniques.
First, scholars study the causes of defects in the laser welding process and continuously improve processing parameters to reduce or eliminate these defects. Second, they explore combining external energy sources, such as magnetic fields and oscillation, with laser energy to improve stability and resolve weld defects, ultimately improving the performance of welded joints.
Laser welding has expanded its field of application from initial heat conduction to current multifield coupling research. Semiconductor lasers have improved their photoelectric conversion efficiency, with lower power consumption and more concentrated light spots, which is the trend in the development of new lasers.
With continued advancements and innovations in laser equipment, laser welding technology is expected to continue to expand its application into more material processing fields, driving the modernization of manufacturing industries.