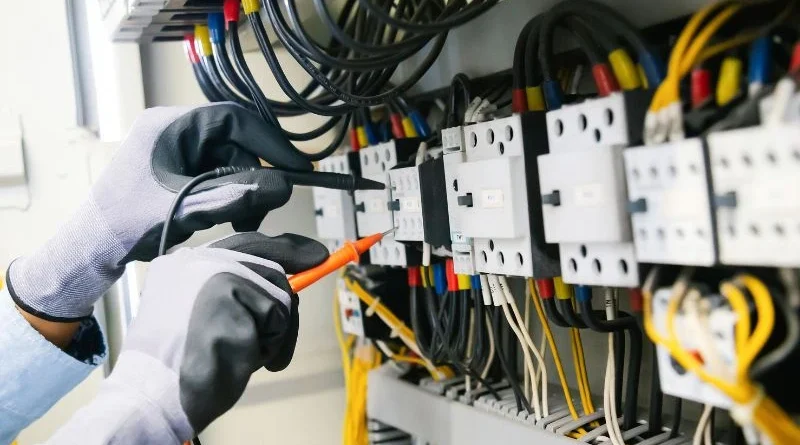
As we journey through the remarkable world of modern technology, it is important to understand the foundations upon which our digital civilization is built. Electrical conductivity in metals and materials is the invisible force that powers our most sophisticated devices, influencing everything from global communications networks to your humble kitchen toaster. This neglected process has fueled the technological renaissance of the 21st century, but it remains a mystery to many. In this blog post, we'll delve into the science behind electrical conductivity, discover its secrets, and examine how it continues to transform our technology landscape. We invite you to join us on this enlightening expedition as we shed light on how electrons move and the future possibilities they offer. Please relax and grab your mental microscope as we begin our journey from simple copper atoms to complex graphene structures!
Electrical conductivity refers to a material's ability to conduct electricity. It is an important property with diverse applications in various industries, such as electronics, telecommunications and power generation. Conductivity can vary greatly between different materials and is influenced by temperature, impurities and magnetic fields. Metals are generally good conductors because they have mobile electrons that can carry electrical charge through the material. Understanding electrical conductivity is crucial in materials research and has implications for the development of new technologies based on conductive materials.
Understanding the Science of Electrical Conductivity
Electrical conductivity is crucial in many areas of our modern world. It refers to the ability of a material to conduct electrical current and allow electrons to flow through the material. Metals are known for their high electrical conductivity, due to the mobile electrons in their atomic structures. These mobile electrons can move easily through the material when an electric field is applied, allowing for the efficient transfer of electrical energy.
Imagine a copper wire connecting the two ends of an electrical circuit. When a voltage is applied to one end, the electrons in the copper can move freely due to the abundance of free electrons, carrying electrical charge through the wire to power devices or generate useful electrical signals.
To understand why metals are such good conductors, we need to look at their atomic structure. In metallic materials, valence electrons separate from their original atoms, forming what is known as a “sea of delocalized electrons”. This sea of electrons is not bound to a specific atom, meaning the electrons are highly mobile and can efficiently conduct electrical charge throughout the material.
However, not all materials have as high an electrical conductivity as metals. Insulators, for example, have extremely low electrical conductivity because they do not have these delocalized electrons. In insulating materials such as rubber or plastic, there are only a few free or moving charges available to carry the electrical current.
- According to a study published in Materials Today, silver has the highest electrical conductivity of any element. It is measured at room temperature at approximately 63 x 10^6 Siemens per meter.
- According to a study published in the Journal of Applied Physics, copper, known for its high conductivity, is just behind silver, with an electrical conductivity value of about 59 x 10^6 Siemens per meter.
- In contrast, plastics are generally strong electrical insulators rather than conductors. For example, Teflon's very low electrical conductivity is about 10^-16 Siemens per meter, according to Polymer International in 2020.
How to measure electrical conductivity
Scientists and engineers use various measurement techniques to quantify and compare the electrical conductivity of different materials. A common method is to measure resistance, essentially the opposite of conductivity. Resistance is measured in ohms (Ω) and indicates the intensity with which a material resists the flow of electrical current.
An ohmmeter or multimeter is the easiest way to measure resistance. By applying a known voltage to a sample of material and measuring the resulting current flowing through it, resistance can be calculated using Ohm's law (R = V/I), where R is resistance, V is voltage, and I is the current.
For example, if a voltage of 5 volts is applied to a sample of material and the resulting current is 1 amp, the resistance will be 5 ohms. This value can then be used to determine the conductivity of the material.
However, it is important to note that measuring electrical conductivity is more complex than measuring resistance. Factors such as temperature, impurities and the presence of magnetic fields can significantly influence conductivity values. Therefore, researchers often take precise measurements and carefully control these variables to obtain accurate and reliable results.
In addition to direct measurement techniques such as resistance, special instruments such as conductivity meters or four-point probes offer more accurate options for determining electrical conductivity. These methods measure electrical conductivity directly in Siemens (S) or microSiemens (µS) units.
Think of these instruments as powerful magnifying glasses that can magnify the behavior of electrons in materials, allowing us to understand their conductivity properties more accurately.
- To measure the electrical conductivity of materials, scientists and engineers often use resistance measurements. A known voltage is applied to a sample of material and the resulting current flow is measured. However, other factors such as temperature and impurities can also affect conductivity, so researchers must carefully control these variables to obtain reliable results. Specialized instruments such as conductivity meters or four-point probe configurations provide more accurate ways of determining electrical conductivity and allow direct measurement on Siemens or micro-Siemens units, allowing a better understanding of a material's conductivity properties.
Comparison between conductors and insulators
When exploring the science of electrical conductivity, it is important to understand the fundamental differences between conductors and insulators. Conductors are materials through which electrical current can flow easily whereas insulators impede or hinder the movement of electrical charge. This difference arises due to variations in their atomic or molecular structure and the behavior of their electrons.
Let's consider a simple analogy to illustrate this concept. Imagine a group of people trying to move along a busy city street. In this scenario, drivers would be like wide highways with multiple lanes, allowing traffic to flow smoothly and efficiently. Isolators, on the other hand, would resemble narrow alleys that force people to walk behind each other, causing traffic jams and slow movement.
The main difference between conductors and insulators lies in their ability to facilitate the movement of electrons. In conductors such as metals, atoms have outermost electrons that are not tightly bound to specific nuclei. These “free” or “delocalized” electrons can move more freely within the material and effectively transfer electrical charge. As a result, metals have high electrical conductivity.
In contrast, insulators have electrons tightly bound to their atomic structures, making it difficult for electrical charge to flow through them. The close bond between electrons and the atomic nucleus prevents the free movement of electrons and inhibits conductivity. Examples of common insulators include rubber, plastic, wood, and glass.
It is important to highlight that there are also materials known as semiconductors, which are intermediate between conductors and insulators in terms of electrical conductivity. Semiconductors have properties that allow them to conduct electricity under certain conditions, although with lower efficiency than conductors.
Now that we understand the difference between conductors and insulators, let's look at the important role that metals play in electrical conductivity.
Role of metals in electrical conductivity
Metals are known for their exceptional ability to conduct electricity, making them indispensable in countless industries. This remarkable property is due to the unique arrangement of electrons in metal atoms and the properties of their electron cloud.
In metals, valence electrons – the outermost electrons involved in bonds – are not confined to a single atom, but form a shared sea of electrons that permeate the entire structure of the crystal lattice. These delocalized electrons are mobile and can move freely through the material under the influence of an electric field.
The high electrical conductivity of metals is due to this sea of mobile electrons. When an electrical potential difference is applied to a metal, the delocalized electrons respond by flowing in a coordinated manner, carrying electrical charge through the material with minimal resistance.
The presence of this sea of electrons gives metals several special properties. First, it allows the efficient transfer of electrical charge from one point to another, making metals ideal for building electrical circuits. Second, metals have low electrical resistance because electrons can easily move through them. This low resistance reduces energy loss in the form of heat during conduction.
It is worth mentioning that the electrical conductivity of metals varies depending on factors such as temperature, purity and alloy composition. The most conductive metals include silver, copper and aluminum, which are commonly used in electrical wiring, power transmission lines and electronic components.
For example, imagine your smartphone without the complex copper wires that connect the various components. Without the excellent electrical conductivity of metals, our modern technology would not work as efficiently as it does today.
Now that we have discovered the important role of metals in electrical conductivity and how they enable efficient charge transfer, let us now explore their practical applications across various industries.
Practical applications in various sectors
The science of electrical conductivity plays a central role in countless industries, enabling advances and innovations that improve our daily lives. Let's explore some practical applications of electrical conductivity in various sectors.
Electrical conductivity is essential for the functionality of electronic devices such as smartphones, computers and televisions. Conductive materials such as copper, aluminum and gold are used in circuits and wires to ensure efficient flow of electricity. Without electrical conductivity, these devices would not be able to transmit data or perform their intended functions.
The automotive industry relies heavily on electrical conductivity in several ways. Electric vehicles require conductive materials to enable power transmission and charging systems. Additionally, advanced sensor technologies utilize conductive materials to enable accurate measurements and diagnostics in modern cars. The integration of electrical conductivity helps improve safety, efficiency and performance in automotive applications.
Electrical conductivity is also of crucial importance in the aerospace industry. Aircraft wiring systems use materials with high electrical conductivity to ensure reliable communication between various components. These conductive materials also play an important role in lightning protection and protect the aircraft from damage during adverse weather conditions. Additionally, electrical conductivity is used in satellite technology to enable uninterrupted communication signals between Earth and space.
In the medical industry, electrical conductivity is used in diverse areas such as diagnostic devices, implantable devices, and electrical stimulation therapies. Conductive materials like silver or carbon are used in electrodes to detect bioelectrical signals for diagnostic purposes. Implantable devices, such as pacemakers, rely on electrical conductivity to deliver precise electrical pulses to regulate heart rate. Electrotherapy techniques use controlled electrical currents to stimulate muscles and nerves for rehabilitation.
Furthermore, electrical conductivity is crucial in the production of renewable energy through solar panels and wind turbines. Conductive materials such as silicon or indium tin oxide allow sunlight to be converted into electricity, facilitating the flow of electrons. Wind turbines also use conductive materials to efficiently transmit the electricity they generate.
These are just a few examples that show the diverse applications of electrical conductivity in different sectors. The importance of this scientific principle cannot be overstated as it enables technological advances that drive progress in our modern world.
Now that we've looked at the practical applications of electrical conductivity, let's look at the development of innovative materials to further improve conductivity.
Development of innovative materials for better conductivity
Innovators and researchers continually strive to develop materials with improved electrical conductivity properties. This pursuit leads to innovative advances and new opportunities for various sectors. Let's explore some innovative approaches to materials development that focus on improving conductivity.
One such area of research includes the use of conductive polymers and additives. These materials offer unique properties compared to traditional conductors such as metals. Conductive polymers offer design flexibility, lower cost, higher density and dimensional stability compared to heavy metal and ceramic parts. By integrating conductive polymers into electronic devices, researchers aim to increase performance while reducing weight and production costs.
Nanomaterials also play an important role in improving electrical conductivity. Graphene, for example, a single layer of carbon atoms arranged in a hexagonal lattice, exhibits exceptional electrical conductivity due to its unique atomic structure. Researchers are exploring methods to integrate graphene into diverse applications, such as batteries, supercapacitors and flexible electronics, to improve their performance and efficiency.
Another interesting area is the study of carbon nanotubes (CNTs) due to their exceptional electrical conductivity. CNTs are cylindrical structures made from carbon sheets laminated to incredibly high aspect ratios. They have excellent electrical conductivity and notable mechanical resistance. Incorporating CNTs into composites or coatings can improve their electrical properties while maintaining other desirable physical properties.
Imagine a future where electronics are more powerful and significantly lighter thanks to the integration of conductive polymers or nanomaterials such as graphene or carbon nanotubes. These innovative materials developments have the potential to revolutionize multiple industries and enable the development of more advanced and efficient technologies.
By exploring innovative materials development strategies to improve conductivity, we gained insight into an important aspect – understanding the effects of temperature, impurities and magnetic fields on conductivity.
Influence of factors such as temperature, impurities and magnetic fields on conductivity
The electrical conductivity of a material is greatly influenced by several factors, such as temperature, impurities and magnetic fields. Understanding how these factors affect conductivity is critical in many industries, including electronics and power generation.
temperature
One of the main factors that affect electrical conductivity is temperature. As a material's temperature increases, its conductivity generally decreases. This is because an increase in temperature leads to increased thermal oscillations of atoms or ions in the material, which can disrupt the flow of electrons. In metals, for example, increased thermal oscillations lead to more frequent collisions between electrons and ions in the grid, resulting in greater resistance and reduced conductivity.
Think of it like trying to navigate a crowded room. When the room is cool and quiet, it is easier to move around freely. However, as more people move around and collide with each other due to warmer temperatures, it becomes more difficult to move easily through the crowd.
impurities
Impurities in a material can also significantly affect its electrical conductivity. Impurities can cause defects or alter the crystalline structure of the material, affecting the free circulation of charge carriers (such as electrons). These defects act as scattering sites for charge carriers, resulting in increased resistance and reduced conductivity. The effect of impurities on conductivity depends on their concentration and type.
material | Conductivity without impurities | Conductivity with impurities |
copper | High | Reduced |
silicon | Low | Strongly affected |
Compare a smooth highway to a road with obstacles like potholes or traffic cones. A clean road allows traffic to flow smoothly and travel faster (increased conductivity), while obstacles impede movement and slow down traffic (decreased conductivity).
Magnetic fields
Another factor that can affect electrical conductivity is the presence of magnetic fields. When a material is exposed to a magnetic field, it can exert an electromagnetic force on charge carriers, affecting their movement. This force can alter the paths of charge carriers, leading to changes in conductivity.
Imagine walking straight ahead while someone pulls you to the side with a magnet. It becomes difficult to maintain the intended trajectory, similar to the way charge carriers in a material are deflected due to a magnetic field.
Understanding the effects of temperature, impurities and magnetic fields on conductivity provides valuable information about how materials behave under different conditions. It also highlights the importance of controlling these factors in various applications, such as: B. electronic devices or power transmission systems.