In recent years, the construction of long-span bridges, super-tall buildings and large sports facilities has increased the demand for H-shaped steel with large size, thick flanges and thick webs.
Hot-rolled H-section heavy steel, with large overall dimensions, flange and web thickness and high stress safety factor, is an ideal material for the construction of long-span bridges and tall steel structures, and is also the focus on research and development at home and abroad.
Related Reading: H Beam vs Steel I Beam

In the mechanical property test of some heavy steel H beams, it was found that there were silvery-white spots of different sizes on the longitudinal tensile and Z-tensile fracture surfaces, and the plasticity of the material decreased significantly.
According to the statistical results of the test data, the number and size of silver-white spots have a great influence on the plasticity of the material.
The larger the size and number of silvery-white spots, the lower the plasticity of the material, but it has no obvious influence on the tensile strength and yield strength of the material, indicating that the silvery-white spots have a direct relationship with the plasticity of the material. material.
To find out the root cause of this effect, researchers from Maanshan Iron and Steel Co., Ltd. and Jiangsu Yonggang Group Co., Ltd. collected samples of heavy H-beam materials with silvery white spots and carried out tensile tests to conduct the physical and chemical inspection and analysis of tensile samples.
1. Physical and chemical inspection
1.1 Macro observation

The tensile fracture surface of the sample (see Fig. 1) was observed macroscopically.
It can be seen in Figure 1 that:
The longitudinal tensile fracture is relatively flat, with little fluctuation and no obvious plastic deformation.
There are silvery-white spots resembling fish eyes on the fracture surface;
The tensile fracture surface in the Z direction fluctuates obviously, but there is no shear lip, radiation area and fiber area.
There are also silvery-white spots resembling fish eyes on the fracture surface.
When observing the longitudinal tensile fracture surface side of the sample (see Fig. 2), it is found that there are a series of micropores and cracks along the tensile direction, and there is a skin warping phenomenon, which is caused by the fracture of the sample pores during the tensile process.

1.2 Analysis of chemical composition
The chemical composition of the sample was analyzed by a direct reading spectrometer, and the chemical composition of the material met the standard requirements.
The hydrogen content of the sample was determined by the hydrogen determination analyzer, and the hydrogen content (hydrogen element concentration) reached 10mg/kg.
Combined with the macromorphological analysis of the fracture surface, it can be preliminarily determined that the silvery-white spots on the fracture surface are hydrogen-induced white spots.
1.3 Metallographic inspection

The metallographic examination and inclusion analysis were performed close to the fracture surface of the sample, and the microstructure was ferrite+pearlite.
The banded structure was not obvious, the grain size was 7.5 to 8.0, and there was no other abnormal structure (see Fig. 3).

Figure 4 shows the microstructure morphology of the defects around the holes on the side of the stressed sample.
It can be seen that the microstructure of the defects around the holes is ferrite + pearlite with signs of deformation, but there is no abnormality compared with the microstructure of other parts, and no obvious coarse inclusions, structural segregation and other abnormal structures are found.
Non-metallic inclusions are classified in accordance with GB/T 10561-2005 Determination of the Content of Non-Metallic Inclusions in Steel by Micrographic Examination of the Standard Classification Chart. The inclusion level is low and no large particle inclusions are found (see Table 1).
Table 1 Non-metallic inclusion levels of samples
Sampling location | Sulfide(A) | Oxide (B) | Silicate (C) | Globular oxide(D) | Single particle spherical | ||||
Fine series | Coarse series | Fine series | Coarse series | Fine series | Coarse series | Fine series | Coarse series | (DS) | |
Table | 0 | 0 | 0.5 | 0 | 0 | 0 | 1.0 | 0.5 | 0 |
Table | 0 | 0 | 0.5 | 0 | 0 | 0 | 0.5 | 0.5 | 0.5 |
Network | 0.5 | 0 | 0 | 0.5 | 0 | 0 | 1.0 | 1.0 | 1.0 |
Network | 0 | 0 | 0.5 | 0.5 | 0.5 | 0 | 1.5 | 0 | 0.5 |
1.4 Analysis of fracture micromorphology


The scanning electron microscope (SEM) was used to observe the longitudinal and Z-direction tensile fracture.
Figures 5 and 6 show the microstructure of fracture due to longitudinal tension and tension in the Z direction, respectively.
There are characteristic flat areas of different sizes on the fracture surface of both fracture types, that is, silvery-white spots observed with the naked eye.
There is a clear boundary between this area and the surrounding area, forming a clear contour with the matrix fracture area.
In the flat area, the morphology of the internal fracture is similar to a tongue, and there are capillary lines in some parts, which have obvious characteristics of brittle fracture.
The fracture shape in this area is mainly a quasi-cleavage fracture.
The appearance of the surface warping defect in the side hole of the tensile specimen is similar to that of tensile fracture, both of which are quasi-cleavage fractures and are essentially the same type of fracture (see Fig. 7).

There are fracture dimples on the entire fracture surface except silvery white spots, which indicates that the silvery white spot area on the fracture surface is a brittle fracture of cleavage fracture, and other areas are still dimple-dominated ductile fractures ( see Fig. 8).

Magnify the area of the silvery-white spot on the fracture surface and note that there are small inclusions in the central area.
The results of the energy spectrum analysis show that the main components are inclusions composed of calcium (see Figures 9,10).

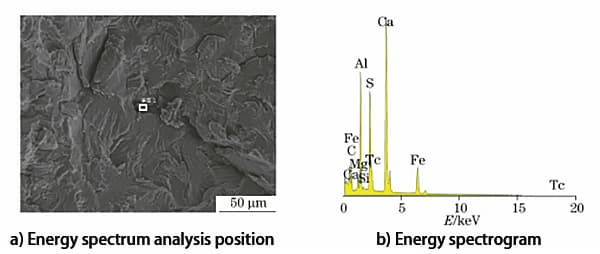
It can be seen from the microscopic morphology of the fracture surface that the silvery-white spot area initiated cracks due to the embrittlement of the matrix and the role of the second phase during the tensile process, and then expanded to fracture, which belongs to induced brittle fracture by hydrogen. .
1.5 Dehydrogenation annealing test
In order to further verify the hydrogen embrittlement behavior of the samples, the same batch of tensile samples was dehydrogenated and annealed at 500℃ for 4h, and then slowly cooled in the oven.
The hydrogen content measured by the hydrogen determiner is 4 mg/kg, which is significantly lower than that before annealing (10 mg/kg).
Table 2 Mechanical Properties of Samples Before and After Dehydrogenation Annealing
Heat treatment | Traction direction | Yield limit/MPa | Tensile strength/MPa | Elongation after fracture/% | Area reduction/% |
Dehydrogenation | Longitudinal tension | 417 | 595 | 22 | – |
Before annealing | Stretch in the z direction | 351 | 574 | – | 33 |
Dehydrogenation | Longitudinal tension | 422 | 602 | 28 | – |
After annealing | Stretch in the z direction | 359 | 582 | – | 49 |
The mechanical properties of the sample before and after dehydrogenation annealing are shown in Table 2.
It can be seen that after dehydrogenation annealing, the plasticity of the sample is significantly improved, but the strength is less improved.
A large number of test results show that the homogeneity of strength and plasticity of samples after dehydrogenation annealing is also significantly improved.

The fracture morphology of the sample after dehydrogenation annealing is shown in Fig.
At this time, there are no silvery-white spots on the micro surface of the fracture, and they are all dimples with uniform size distribution, indicating that the sample fracture is a ductile fracture.
2. Comprehensive analysis
The heavy H beam will inevitably be invaded by hydrogen from atmospheric water vapor, water in ore or alloy, and rust in scrap during smelting and rolling.
The destruction mechanism of hydrogen in steel is: Because the solubility of hydrogen in liquid steel is much higher than that in solid metal, the hydrogen in liquid metal remains in the metal before it can escape during the solidification of H-beam heavy steel during casting, resulting in the continuous diffusion and aggregation of hydrogen into the material.
When local aggregation reaches a certain content, it will cause white spots, hydrogen bubbles and other phenomena.
The local aggregation of hydrogen in steel makes the material brittle, load capacity and plasticity decrease.
The H-shaped heavy steel flange and web are 67.6mm and 42mm thick respectively, so hydrogen is more difficult to diffuse and escape, accumulating in the center, and is more likely to occur hydrogen embrittlement.
Generally, hydrogen accumulates in parts with serious defects, such as inclusions, carbides, micropores, etc.
In the defect, hydrogen atoms combine into hydrogen molecules, which generate obvious stress and form hydrogen bubbles.
In the process of longitudinal and Z-direction tension, these defects are located on the surface of the heavy H beam, and the hydrogen bubble is not consistent with the deformation of the matrix, resulting in the rupture of the bubble, so that the skin deformation defect is formed on the side of the tensile specimen.
3. Conclusions and suggestions
The reason for the abnormal tensile fracture surface and decreased plasticity of heavy H-beam is that the hydrogen content of the material is too high, resulting in hydrogen embrittlement.
Hydrogen embrittlement can be eliminated by dehydrogenation annealing, which belongs to reversible hydrogen embrittlement.
To avoid such problems, it is suggested to strengthen the management of raw materials for steelmaking to prevent the invasion of external hydrogen due to the moisture of raw materials.
In the smelting process, the vacuum circulation degassing furnace can be used to optimize the vacuum process route to make the initial hydrogen escape from the molten steel, so as to reduce the hydrogen content in the molten steel.