Screws are a commonly used fastener. If a screw breaks even slightly, it will need to be disconnected for maintenance. However, if it breaks badly, it can cause damage to machines and even cause human deaths.
Merely replacing a broken screw does not completely eliminate the risk of further breakage. Therefore, it is essential to analyze the factors that contribute to screw breakage and improve them.
From an individual perspective, the factors that lead to screw fractures can vary widely. However, by examining a large number of screw fracture samples, some common features can be identified.
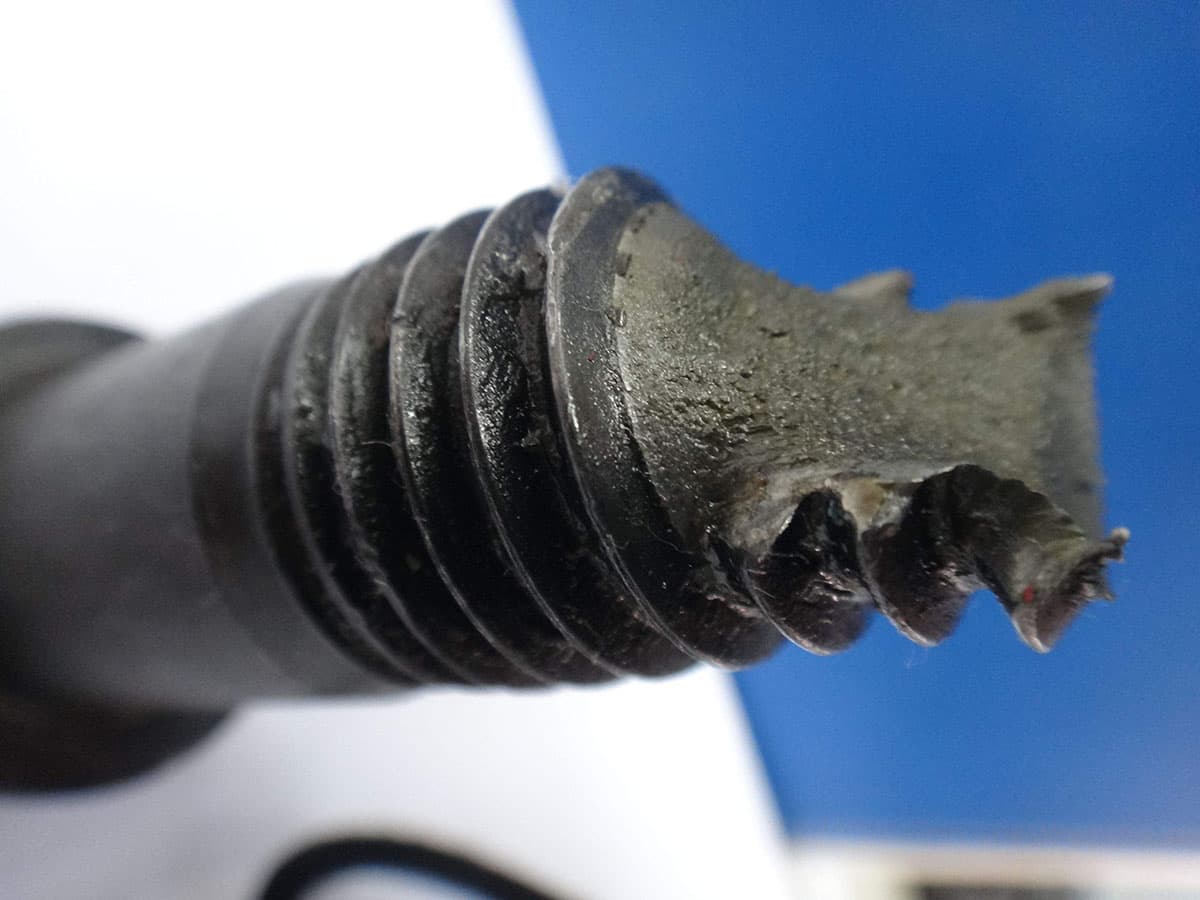
1. Phase characteristics and correlation analysis of factors influencing screw fracture
The life cycle of screws can be divided into four stages: design, manufacturing, use and maintenance, with failure being a possible result. Interruption cracks are the most damaging type of failure.
To prevent screw fracture failure, it is essential to understand the complete set and subset of factors that influence screw fracture at different stages of its life cycle.
Table 1 and Table 2 are obtained after statistical analysis of the factors that influence the fracture of 227 screws.
Table 1 Statistical analysis of factors affecting fracture in staged screw fracture samples
Total | 227 | |
Single Stage Factor Breakdown | Manufacturing | 81 |
Project | 34 | |
Materials science | 14 | |
To use | 14 | |
Combination of two-stage factors causes breakage | Design + Manufacturing | 27 |
Manufacturing + Materials | 17 | |
Manufacturing+use | 14 | |
Design + use | 7 | |
Design+Materials | 1 | |
Use+Material | 1 | |
Broken by the combination of 3 stages + material factors | Design+Manufacture+Use | 7 |
Design+Material+Manufacturing | two | |
Material+Manufacture+Use | 7 | |
3 steps + materials | 1 |
Table 2 Correlation Analysis of Three-Stage Screw Fracture and Materials in Screw Fracture Samples
Correlation Classification | Proportion/% | ||
Manufacturing related | independent | 81 | |
combination | 75 | ||
Total | 156 | 68.7 | |
Design-related | independent | 34 | |
combination | 45 | ||
Total | 79 | 34.8 | |
Material-related | independent | 14 | |
combination | 29 | ||
subway | 43 | 18.9 | |
Usage-related | independent | 14 | |
combination | 37 | ||
Total | 51 | 22.5 |
Screw fracture can be caused by a single factor or a combination of multiple factors.
Table 2 shows that 77.5% of screw fracture samples are caused by pre-use factors, while 68.7% of screw fractures are manufacturing related.
2. Factors and mechanisms that affect fracture at different stages of screw life
There are several factors that can affect the life of screws, including their susceptibility to fracture.
However, due to space constraints, we will only perform a preliminary analysis of mechanisms that have a high frequency of influence.
2.1 Factors and mechanisms affecting screw fracture in the design phase
According to Table 3, the main factors responsible for bolt fracture during the design phase are inadequate material selection, external forces acting on bolts, bolt structure design, small diameter, and difference temperature caused by the use of different materials. These five factors alone account for 85 instances, which is equivalent to 82.5% of the total of 103 instances observed in the design phase.
2.1.1 Effect of inappropriate material selection on screw fracture
Material selection is a crucial aspect of screw design. Table 4 demonstrates the components impacted by inappropriate material selection in screw fracture samples.
Environmental and stress corrosion, insufficient or excessive material strength and inadequate heat treatment processes were the cause of 47 of the 58 incidents, representing 81.0% of cases.
Table 3 Classification and frequency of factors affecting screw fracture in the design phase in screw fracture samples
Affected items | 103 |
Inadequate selection of materials | 42 |
External influence: vibration and insufficient rigidity of connectors | 17 |
Screw Structure Design | 14 |
Small diameter | 7 |
Temperature difference load caused by different materials | 5 |
Number and arrangement of screws | |
Anti-loss | 3 |
Length and connection method | 3 |
Inadequate design requirements | 3 |
other | 5 |
(1) Impact of environment and stress corrosion cracking on bolt fracture
Stress corrosion is a failure phenomenon that occurs in materials when they are subjected to a combination of static stress (especially tensile stress) and corrosion.
When a screw is exposed to stress and a corrosive environment, the surface oxide film of the screw can be damaged due to corrosion. The damaged surface becomes the anode and the undamaged surface becomes the cathode.
As a result, the anode current density increases dramatically, causing additional corrosion of the damaged surface. Under the influence of tensile stress, cracks gradually appear in the damaged area, which gradually expand until the screw ruptures.
To avoid stress corrosion, it is important to choose materials with strong resistance to it. For example, in environments containing high temperature water with sulfides, it is recommended to use chrome-nickel austenitic stainless steel with a low manganese content.
Furthermore, the bolt structure design must be optimized to reduce stress concentration.
Improving the corrosion environment can also help prevent stress corrosion cracking. This can be done by adding corrosion inhibitors to the corrosive medium or using a metallic or non-metallic protective layer to isolate the corrosive medium.
(2) The influence of poor screw material performance on screw fracture.
Material performance covers multiple indicators, and poor performance occurs when the selected bolt material is incompatible with the service environment. Using materials beyond their engineering capability may lead to screw fracture.
For example, the coupling bolt of a 200 MW unit fractured due to the original use of 35 steel that was not quenched or tempered and had a coarse widmanstatten or banded structure. Upon analyzing the broken bolt, it was concluded that 35 steel was not suitable for manufacturing the coupling bolt. Instead, 40CrNiMo steel was used to improve the screw material's comprehensive mechanical properties.
However, it is crucial to ensure the hardness of screws and coupling materials match when using 40CrNiMo steel. When examining the broken screw, it was found that the frictional wear of the screw with a hardness of (260 ~ 280) HB caused damage to the screw hole. Therefore, the use of 40CrNiMo steel to improve screw hardness and achieve high fatigue strength may have adverse consequences.
Consequently, it is necessary to perform a comprehensive mechanical properties test to obtain low notch sensitivity, corresponding hardness and flexural fatigue resistance of the screw before transitioning to its use.
(3) Impact of excessive or insufficient strength on screw fracture
It's easy to understand that insufficient strength can cause screw fractures, but it's easy to ignore the relationship between excessive strength and screw fracture.
High-strength screws not only increase sensitivity to notch stress concentrations, but also exhibit greater susceptibility to hydrogen embrittlement.
Hydrogen-induced cracking typically occurs when the hydrogen content in steel exceeds 5 parts per million (ppm). However, with high-strength steel, hydrogen atoms in the lattice holes will concentrate at stress concentration sites generated by notches through diffusion, even when the hydrogen content in the steel is less than 1 ppm.
These hydrogen atoms interact with the dislocations, causing the dislocation lines to become stuck and unable to move freely, making the body brittle.
2.1.2 External factors affect screw fracture
(1) Effect of vibration on screw fracture
The vibration response of the connecting screw is mainly influenced by two factors: the modal characteristics of the connecting screw and the vibrational excitation transmitted to the screw by the connecting part.
After the connection screw between a transmission and a power take-off broke, a modal test was carried out on the long screw. The results showed that, under the clamping torque condition of 45 N·m, the natural frequency of the first bending mode was 1155 Hz and the modal damping ratio was 0.67.
During the vibration response test of the transmission power take-off under engine operating conditions, it was observed that when the transmission system was in operation, the long screw was excited by significant vibration with a main vibration frequency of 1000 to 1500 Hz .The first bending frequency of the long screw was within this frequency range and the damping rate was very low.
This caused a resonance amplification effect, resulting in a significant bending resonance response of the screw and a high dynamic bending stress in the threaded connection. As a result, the connecting bolt broke prematurely.
(2) Impact of insufficient rigidity of connected parts
Insufficient rigidity in the connected parts not only generates vibrations, but also causes uneven stresses on the screws.
Anchor bolts on a marine diesel engine broke quite often. The results of the analysis revealed that the main engine presented large vibrations, mainly vertical vibrations caused by the weak rigidity of the base – bilge.
After the wedge positioning block of the host positioning bracket was firmly welded, the anchor bolt no longer broke as its rigidity was strengthened.
Both ends of the steel plate of the drum housing of a hoist are connected to the flange plate with M22 bolts. However, there is no reinforcing support ring or circumferential casing ring inside the drum, which creates a simply supported beam along the axis.
As a result, the rigidity is poor, and under working conditions, the middle of the drum shell undergoes the greatest deformation, placing the M18 connecting bolt under maximum force and causing it to break. Meanwhile, the connecting bolts near the flange plate at both ends of the drum did not break.
2.1.3 Effect of screw hole structure on screw fracture
The main factor contributing to bolt fracture in bolt frames and bolt holes is a small transition thread. This includes the transition thread at the thread root, screw and screw head, and counterbore. A small transition fillet not only generates stress concentration, but also leads to the generation of large internal stresses during heat treatment, resulting in the appearance of microcracks or cracking tendencies that reduce the bolt's load capacity.
The combination of external loads and internal stresses causes the bolt to bear a load that exceeds its limit, leading to breakage.
For example, the main bearing screw of a DF 7B locomotive diesel engine was broken due to this phenomenon.
After the modification, the central screw hole was eliminated, resulting in a 45% increase in the support area of the main support screw head and a substantial improvement in the strength of the threaded part.
Furthermore, the elimination of the inner hole of the screw eliminated the stress concentration caused by the thread structure of the inner hole, resulting in an increase in the fatigue strength of the screw.
2.2 Factors and mechanisms that affect screw fracture in the manufacturing phase
The quality of heat treatment, machining, processing transition fillet size, fitting and assembly, and the screw forming process are the main factors that affect the risk of screw fracture during the manufacturing phase.
There are 141 factors, representing 89.2% of 158. See Table 5 and Table 6.
Table 5 Classification and Items of Factors Affecting Screw Fracture in the Manufacturing Stage
Item | 158 |
Heat treatment quality | 71 |
Machining quality | 40 |
The fillet is very small | 11 |
Fit and assembly quality | 10 |
No integral defects in the forging or forming process | 9 |
Defect in the root surface of the screw tooth | two |
Lining fracture and corrosion | 1 |
other | 14 |
Table 6 Classification and items of factors that affect screw fracture due to heat treatment
Item | 87 |
Heat Treatment Process Design and Process Quality | 33 |
hydrogen embrittlement | 14 |
Decarburization and partial overburning | 11 |
Dysplasia | 8 |
High hardness and low plasticity | 6 |
Surface or central carburetion | 5 |
Quenching quality and pretreatment intelligence | 5 |
Heat treatment and material conflict fixing leather rope | 1 |
others | 4 |
2.2.1 Effect of heat treatment on screw fracture
The main factors responsible for screw fracture are heat treatment process design and process quality, hydrogen embrittlement, decarburization, local excessive burning, poor structure, high hardness and low plasticity. These factors collectively account for 82.8% of the 87 cases studied.
(1) Effect of heat treatment process design and process quality on screw fracture
An example is presented to demonstrate the impact of inadequate heat treatment processes on screw fracture. When hypoeutectoid quenched and tempered 42CrMo steel is used as the screw material and the section size is large (e.g. ≥ 500 mm), achieving the yield rate of 0.9 with traditional quenching and tempering treatment becomes a challenge.
To achieve the goal, it is necessary to reduce the tempering temperature, that is, use tempering at a medium temperature or tempering at a lower temperature. However, this would result in decreased toughness, increased strength, and the metallographic structure would contain defects (the second type of temper brittleness).
The test results of a batch of screws are as follows: high strength (σb>1200 MPa), high hardness (HBS>400), and the metallographic structure is tempered troostite, which fully supports this observation.
Studies carried out nationally and internationally indicate that the greater the resistance of steel, the more prone it is to cracking. Therefore, if the toughness is insufficient when σb > 1200 MPa, low stress brittle fracture can occur easily.
(2) Effect of hydrogen embrittlement on screw fracture
Screws used in corrosive environments and requiring high strength often need anti-corrosion treatment.
However, some anticorrosive processes, such as chrome plating, can lead to hydrogen embrittlement.
Research has shown that the greater the strength of the material, the more sensitive it is to hydrogen embrittlement and the faster cracks will grow.
In steel microstructures, susceptibility to hydrogen embrittlement generally follows this order from highest to lowest: martensite, upper bainite, lower bainite, sorbite, pearlite, and austenite. High strength depends on the corresponding metallographic structure.
Hydrogen embrittlement can result from internal or external hydrogen. Internal hydrogen is generated during manufacturing, while external hydrogen penetrates during use.
Generally, internal hydrogen will cause the bolt to crack or break before or after use, while external hydrogen requires a build-up process to reach the level of damage that will cause the bolt to fracture.
Therefore, it takes time for the screw to break.
To prevent hydrogen embrittlement fractures caused by electroplating, it is recommended to use hydrogen embrittlement-free coatings such as zinc-chromium coatings commonly used in industries such as automotive and aerospace.
2.2.2 Effect of machining quality on screw fracture
During the screw manufacturing process, defects such as wrinkles, folds and microcracks can form due to improper processing. These defects often lead to further cracking or expansion of screw threads during rolling, forming or heat treating. In particular, such defects tend to be concentrated at the root of screw threads.
Under tension or cyclic loading, microcracks at the root of bolt threads are susceptible to stress concentration, which can trigger sources of fatigue and cause fatigue fractures from multiple sources.
On a 350 MW gas turbine unit, a machining stripe was observed on the fracture surface of the heater bolt. This strip was located at the junction of the screw and screw head. Additionally, a large pit of corrosion was found in the strip, indicating obvious corrosion in the crack before the bolt broke.
Further appearance inspections revealed that the surface of the bolt's polished shank was rough. This not only became the source of stress concentration, but also provided conditions for crevice corrosion and stress corrosion.
2.3 Analysis of material factors and frequency affecting screw fracture
Table 7 displays the influencing factors and material-related items that contribute to screw fracture in the sample of broken screws. It includes 39 items related to inclusions, material quality, metallurgical defects and excessive content of chemical elements, which account for 86.7% of the total of 45 items.
Table 7 Factors and Items that Affect Screw Fracture by Materials
Item | 45 |
Inclusion | 16 |
Quality of materials | 10 |
Metallurgical defect | 7 |
Chemical elements of materials | 6 |
Segregation | 4 |
Microcrack | two |
(1) Influence of inclusions on screw fracture
When magnesium and calcium from foreign inclusions, as well as sulfur, manganese, chromium and other elements in the material, segregate toward the grain boundary, it can cause grain boundary embrittlement. This embrittlement can occur in local areas, leading to possible cracks.
Furthermore, if the size of inclusions in bolts is excessively large, especially near the surface layer, it can accelerate the initiation and propagation of fatigue cracks in bolts.
The banded distribution of MnS inclusions in steel can also increase its susceptibility to hydrogen-induced cracking.
(2) Effect of supplied material properties on screw fracture
In certain service environments, it is not enough to focus solely on the strength and hardness of screw materials. Comprehensive consideration must be given to factors such as plasticity, impact resistance, corrosion resistance, notch sensitivity and the difference in performance between ambient temperature and working temperature.
Material performance nonconformity refers to a situation where the material supplied does not meet project requirements.
After the gas heater bolts of a gas turbine unit broke, it was discovered through chemical composition and metallographic analysis that the broken bolts were not made of the type 304 stainless steel specified in the design. Instead, they were cast after several different stainless steel materials were melted again, and their corrosion resistance was inadequate, leading to galvanic corrosion between the screws and the blank plate due to the different electrode potentials at the beginning.
Although the broken bolts on the flange of the intermediate pressure regulating valve of a steam turbine generator passed the spot checks for mechanical strength and impact resistance at room temperature, both failed to qualify the test at the operating temperature of 540℃.
(3) Influence of metallurgical defects of materials on screw fracture
The presence of gaps, bubbles, slag inclusions and internal cracks in the bolt materials significantly decreases the actual allowable stress of the materials.
Macro and micro analysis of the fracture surface of high-strength screws shows that after the initiation of fracture at the origin of the crack, the process expands quickly and unstable until it breaks. This is because the material contains numerous microdefects, such as microcracks and micropores, which reduce the actual allowable stress and are also a prerequisite for rapid and unstable crack growth.
The formation of these microcracks is related to incomplete outgassing and slagging during casting, as well as incomplete elimination during subsequent forging.
2.4 Analysis of service and frequency factors affecting bolt fracture
The main factors contributing to screw fracture during use include pre-tightening force, uneven tightening force, improper tightening methods, installation problems and other related problems.
Of the 92 recorded occurrences, these three problems were responsible for 69 occurrences, corresponding to 75.0% of cases, as shown in Table 8.
(1) Effect of pre-tightening force on screw fracture
The sealing structure of a clamp connection is inaccessible due to high temperature and high pressure conditions.
To tighten the fixing screw, the operator uses a special wrench approximately 1 meter long until it can no longer be tightened. However, this causes the bolt preload to exceed the allowable bolt tension.
When the pipeline pressure increases, the bolt tension increases further, resulting in the bolt breaking in a short time.
In the case of a group of connecting rod bolts, if a bolt does not have sufficient preload, a large gap will form between the connecting rod journal and the bearing bushing.
Under high-speed crankshaft operation, the bolt will bear large impact loads and alternating bending moments, leading to fatigue and fractures. The load must then be transferred to another bolt, which will also be overloaded and fracture.
(2) Uneven clamping force and inappropriate clamping method
During compressor maintenance, maintenance personnel did not use a torque wrench to apply the designed pre-tightening force to the bolts. Instead, they used a solid wrench and sledgehammer to apply force, relying only on their experience to estimate the correct amount of pressure.
As a result, the pre-tightening force applied to the screws was inconsistent. Bolts located in areas convenient for hammering received greater pre-tightening force, while those in less accessible areas received less force.
After analyzing the fracture distribution of compressor head bolts, it was found that most of the bolts located in the convenient place for hammering had broken, which is consistent with the analytical results.
3. Conclusion
(1) Quality objectives for the life cycle of mechanical products are based on achieving life cycle quality for all parts, including screws. An innovative approach is to establish the concept of life cycle quality at the part level and identify the factors that influence screw fracture.
(2) The life cycle quality of screws is influenced by various stages and factors. The establishment of a set of factors that affect screw fracture can assist in general planning and selection of these factors, thus achieving the quality objectives of the screw life cycle.
(3) There is a need to continuously improve the set of factors that influence screw fracture, as well as supplement and revise relevant teaching materials and documents to support the practice of the life cycle quality concept for screws and mechanical products.