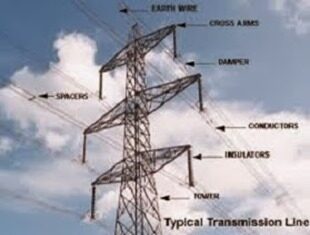
In a constantly evolving world, driven by the desire for reliable and efficient power distribution, mastering transmission lines has emerged as a crucial art. “Wiring the Future: Mastering the Art of Transmission Lines” summarizes the central role these complex networks play in shaping our modern world. As societies become increasingly dependent on electricity in all areas of life, from households to industries, the science behind the continuous flow of electricity is taking center stage. This exploration delves into the complexities of transmission lines, their critical importance in meeting the growing demand for electrical power, and the innovative measures being taken to optimize their design and functionality. Join us as we unravel the complexities of this essential art that electrifies our future.
Economic Transmission Tower Design
Transmission line towers account for approximately 28% to 42% of the transmission line cost. The growing demand for electrical energy can be met more economically through the development of modified lightweight construction models of transmission line poles. Choosing an ideal contour arranged with the correct height, support system, cross-arm type and additional parameters provides broad scope in developing a cost-effective transmission tower design. The goal of every designer is to plan ideal systems. However, due to practical limitations, this was achieved through intuition, practice and repeated attempts.
Factors Affecting Tower Cost
The cost of aerial transmission towers is influenced by a number of factors that play a critical role in determining the overall cost of installing and maintaining these essential infrastructure components. Understanding these factors is essential to make informed decisions that contribute to the design and implementation of cost-effective towers. The main factors affecting tower cost include:
used material
The choice of materials for the construction of catenary poles has a direct impact on costs. Poles can be made from materials such as steel, concrete and composites. The cost of these materials, their availability and their suitability for specific environments contribute to the overall cost of the mast.
Tower height
Tower height is a significant cost factor, as taller towers require more material and technical effort. Tall towers are necessary for traversing difficult terrain or maintaining safe distances from obstacles such as buildings and trees, but they also increase costs due to increased material and construction requirements.
Project complexity
The complexity of the tower design, including the number of legs, cross arms and bracing systems, can impact costs. More complex projects often require specialized engineering and manufacturing work, resulting in higher costs.
Land and location
The geographic location and terrain where the transmission line is installed can greatly affect the cost of the tower. Difficult terrain, such as mountainous regions or areas with difficult soil conditions, may require custom mast designs and more extensive construction, resulting in higher costs.
Transport and installation
The logistics of transporting tower components to the installation site and assembling them can significantly increase the overall cost. Difficult to access locations may require specialized equipment or additional effort, increasing transportation and installation costs.
Regulations and licenses
Complying with local regulations, environmental impact assessments, and obtaining necessary permits can increase project administrative costs. Compliance with these requirements ensures that the towers are installed safely and in accordance with legal standards.
Maintenance and durability
The long-term costs of tower ownership include maintenance and repair costs. Towers designed for durability and ease of maintenance may have a higher initial cost, but can save money over the life of the tower due to reduced maintenance requirements and downtime.
Project scope
The scope of the transmission line project, including the number of poles required, can impact the cost. When manufacturing and installing multiple poles, economies of scale can come into play, resulting in potential cost savings.
Innovations and technology
Incorporating innovative design concepts and technologies can impact costs. Although advanced materials and design techniques may increase initial costs, they can provide long-term benefits by reducing maintenance needs or improving tower performance.
Causes and effects of power grid outages
Common causes of errors
Failures can occur in power systems from a variety of sources, interrupting the normal flow of electricity and potentially causing damage to equipment and infrastructure. Understanding these common causes of failure is critical to maintaining a reliable and efficient power distribution network. The main causes of errors include:
Short circuits
-
- Phase-to-ground error: Occurs when a conductor comes into direct contact with the ground, often due to insulation failure or accidental contact.
- Phase-to-phase error: Occurs when two or more conductors of different phases come into contact, resulting in a short circuit between these phases.
Open circuits
-
- Broken ladder: Mechanical damage or wear and tear can cause a conductor to break, thus interrupting the flow of current.
- Loose Connections: Loose or corroded connections in electrical components can cause open circuits and interrupted power flow.
Insulation failure
-
- Aging and Degradation: Over time, the insulating materials used to separate conductors can degrade due to environmental influences, temperature fluctuations and electrical stress.
- Environmental Factors: Extreme weather conditions, pollution and humidity can contribute to the deterioration of insulation.
Device malfunction
-
- Transformer failures: Transformer failures, such as winding shorts or insulation breakdowns, can interrupt power distribution.
- Failures in switchgear and circuit breakers: Defective protective devices can result in failure to isolate defective parts of the system in the event of a failure.
Lightning strikes
-
- Lightning strikes can directly strike power lines or nearby buildings, causing temporary surges that lead to outages.
Human error
-
- Errors in device maintenance, repair, or installation may inadvertently cause short circuits, interruptions, or other errors.
Animal interference
-
- Animal contact with power lines can cause short circuits or interruptions in electrical circuits and therefore malfunctions.
Device Overloads
-
- Operating electrical equipment beyond its designed capacity can result in overheating, breakdown of insulation, and ultimately malfunction.
Natural events
-
- Earthquakes, hurricanes and other natural disasters can damage electrical infrastructure and cause disruptions.
Effects of failures on power systems
Failures in power systems can have significant and far-reaching consequences that affect the stability, security and reliability of the power supply. Understanding the impact of failures is critical to developing robust systems and implementing appropriate mitigation strategies. The effects of power system failures include:
Power supply interruption
-
- Disturbances can lead to sudden interruptions in power supply, causing disruption to consumers, businesses and industries.
- Disruptions in critical sectors such as healthcare, communications and transport can have serious social consequences.
Voltage instability
-
- Disturbances can lead to imbalances and voltage fluctuations in the network and thus affect the quality of the energy supply.
- Overvoltages or undervoltages in the event of a fault can damage sensitive devices and systems.
Current overheads
-
- Short circuits and faults can lead to excessive currents in the system, which can lead to overloading of lines and devices.
- Overcurrents can cause damage to equipment, increase system losses and pose a risk of fire.
Equipment damage
-
- High residual currents can damage transformers, circuit breakers, switches and other electrical components.
- Damaged equipment may need to be replaced or repaired, resulting in maintenance costs and downtime.
Fire risks
-
- Faults with high currents can generate heat and sparks, posing a fire risk to surrounding infrastructure and buildings.
- Fire incidents can result in property damage, economic losses and safety concerns.
Safety risks for personnel
-
- Errors can create dangerous conditions for workers and technicians performing repair or maintenance work.
- People's safety can be endangered by electrical shocks and the effects of high residual currents.
Impact on the transmission and distribution system
-
- Failures can trigger cascading effects, where a single failure in one part of the system causes subsequent failures in other parts, potentially resulting in widespread outages.
- Voltage fluctuations and instabilities can affect transmission lines and neighboring distribution networks.
Industrial and commercial consequences
-
- Fault-related power outages can lead to production stoppages in industry and commerce, thus affecting productivity and sales.
- Financial losses may occur due to operational interruptions and delays in project delivery.
Live effects
-
- Power outages caused by outages can cause disruption to residential customers and impact their daily activities and routines.
- Damage to electronic devices and components caused by voltage spikes in the event of failure can result in repair or replacement costs.
Ecological damage
-
- Outages caused by outages can impact critical infrastructure such as water treatment plants, causing potential environmental problems.
Three-legged tower
Generally, 4-legged lattice towers are used as transmission towers. Three-legged towers are only used for radio, microwave, guyed towers and telecommunications, but not in the energy sector as transmission towers. The 3-legged transmission tower models are very robust because the arrangement of the crossarms and supports is not possible easily and perfectly. Axial force and deflection are increased in 3-leg tower modules compared to 4-leg tower modules, but can be converted to a steel weight of 21.2%, which is created when using a 3-leg tower in conjunction with a 4-legged tower. leg Use tower.

Benefits
- Simplicity: The three-legged design offers a simpler structure with fewer components compared to other tower types.
- Aesthetics: The triangular configuration can be visually appealing and blend well with the environment.
- Lower wind load: Aerodynamic design offers less wind resistance, making it suitable for areas where strong winds occur.
species
- Self-supporting towers
-
- Independent towers that do not depend on support cables.
- Suitable for relatively flat terrain and shorter spans.
- Guyed towers
-
- The towers are stabilized by guy wires attached to anchors in the ground.
- Used for taller structures and areas with uneven terrain.
Components
- Legs: Three vertical legs serve as the main support for the transmission lines.
- Crossed arms: Horizontal arms hold the transmission lines and insulators.
- Tension: Diagonal braces provide structural integrity and improve stability and load distribution.
- Foundation, donation: Anchoring system that fixes the tower to the ground.
Design considerations
- Height: Determined by factors such as span, terrain and clearance requirements.
- Load Capacity: Designed to withstand environmental stresses such as wind, ice and electrical forces.
- Material: It is often made of steel due to its strength and durability.
- Terrain: Suitable for various terrains, including plains, hills and some mountainous regions.
Forms
- Three-legged masts are used on medium and high voltage lines.
- It occurs in energy distribution networks and connects energy generation sources to substations and consumers.
Progress
- The focus of ongoing research is on optimizing tower designs in terms of structural efficiency, cost-benefit ratio and environmental aspects.
- Advances in materials and manufacturing techniques are contributing to more durable and resilient tower structures.
Four-legged tower (power line pylon)
Transmission towers are used to connect high voltage or high current transmission lines. This is generally due to the need for support structures of moderate dimensions. The Design Engineer will have the exciting task of designing and constructing transmission structures to withstand large conductor loads in exposed, high-temperature weather conditions and to protect the public to ensure satisfactory operability.
Benefits
- Stability: Four-legged construction provides a solid foundation and provides good stability against wind, ice and other environmental stresses.
- Load distribution: Load capacity is evenly distributed across the four legs, reducing the load on individual components.
- Easy maintenance: Access to equipment and ladders is relatively convenient due to the open structure design.
- Versatility: Suitable for various terrains and transmission line configurations.
- Cost Effective: Repetitive design and balanced load speed manufacturing and construction.
species
-
Self-supporting towers:
- These towers are freestanding and do not require additional support from guy wires.
- Commonly used on flat terrain or areas where the use of guy wires is impractical.
-
Guyed towers:
- These towers are stabilized by guy wires attached to anchors in the ground.
- Used in areas with uneven terrain or where taller structures are required.
Components
- Legs: Four vertical legs serve as the main support for the transmission lines.
- Crossed arms: Horizontal arms hold the transmission lines and insulators.
- Tension: Diagonal braces provide structural integrity and improve stability and load distribution.
- Foundation, donation: Anchoring system that fixes the tower to the ground.
Design Considerations
- Height: Determined by factors such as span, terrain and clearance requirements.
- Load Capacity: Designed to withstand various environmental stresses, including wind, ice and electrical forces.
- Material: Typically made of steel due to its strength and durability.
- Terrain: Suitable for a variety of terrains, including plains, hills and mountains.
Forms
- Four-legged masts are used on high and extra-high voltage lines.
- Commonly used in energy distribution networks, connecting energy generation sources to substations and consumers.
Progress
- The focus of ongoing research is on optimizing tower designs in terms of cost-benefit, structural efficiency and environmental impact.
- Advances in materials and manufacturing techniques are leading to more durable and resilient tower structures.
Conclusion
In short, the journey through the world of transmission lines reveals a tapestry of innovation, engineering and unwavering commitment to providing a constant flow of power in a dynamic world. “Wiring the Future: Mastering the Art of Transmission Lines” highlighted the critical role these networks play in shaping the technology landscape. As we face the challenges of an energy-hungry future, transmission line design optimization is proving to be a cornerstone that ensures reliability, sustainability and savings. With an unwavering focus on efficiency, safety and adaptability, the art of transmission lines will continue to evolve, connecting us to the energy that drives progress and lighting the path to a brighter, electrified future.