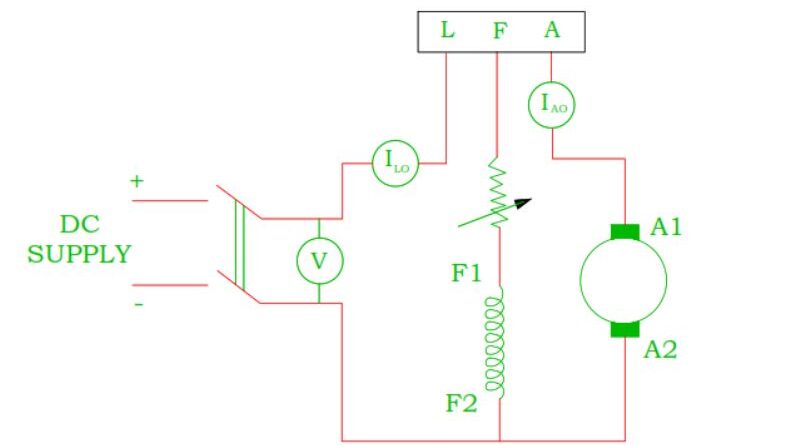
The quest for efficient use of energy in electrical engineering is a driving force behind technological advances. A key tool that represents the epitome of precision in this effort is the Swinburne test – a sophisticated method that unlocks the secrets to the efficiency of DC machines. This method sheds light on machine performance and takes predictive analytics to a new level.
The essence of the Swinburne test
Basically, the Swinburne test revolves around the delicate interplay between idling and the complexities of losses in a DC machine. Operating a generator or DC motor at idle opens opportunities for investigations that go beyond traditional efficiency assessment. A fascinating journey of measurement and evaluation begins when the machine comes to life without external gears.
Act in the void
The direct current machine, be it a generator or a motor, assumes the role of an engine at this point. This idle operation is the canvas on which the portrait of efficiency is painted. When the machine runs without load, it reveals the inherent losses.
Highlighted losses
During this silent operation, the machine's inherent losses come to the surface. These losses include core hysteresis, eddy current losses, and the ever-present friction and air losses. The Swinburne test highlights these normally invisible culprits, revealing their role in the machine's overall performance.
Mapping efficiency
With knowledge of these losses, engineers can go beyond idle operation and predict efficiency under various load conditions. The test provides interesting insight into the machine's potential efficiency in various operating scenarios.
Meaning and applicability
It is important to note that the Swinburne test is not a one-size-fits-all solution. This method applies to DC machines that maintain a constant flow under different operating conditions. These machines include DC shunt machines and DC compound machines.
Improved Efficiency Analysis
The Swinburne test pushed the limits of traditional testing methods. It offers engineers a powerful tool to measure efficiency and predict machine behavior under various workloads. This predictive capability promotes a deeper understanding of machine dynamics and enables informed decisions to optimize energy usage.
Efficiency of DC machines through no-load loss method

Brilliant idle performance
The no-load loss method involves operating a generator or DC motor at no load. This special operating condition exposes the losses inherent to the machine. If the device operates without external limitation, losses that occur without mechanical work can be measured.
Understanding Losses
During this idle operation, the machine suffers losses due to various factors. These losses include core losses (hysteresis losses and eddy currents) as well as friction and air resistance losses. Each of these elements contributes to the overall energy inefficiency of the machine.
Predictive power
What makes the no-load loss method particularly powerful is its predictive power. By quantifying losses under idle conditions, engineers gain insight into how the machine behaves under different load conditions. This predictive capability enables informed decisions to optimize efficiency.
Applicability and scope
The no-load loss method is most effective when applied to DC machines that maintain a constant magnetic flux throughout their operating range. This method finds its ideal basis in devices such as DC shunt and compound machines, where the change remains constant regardless of different operating loads.
Improving efficiency analysis
This method goes beyond the traditional limits of efficiency assessment. It sheds light on the often overlooked losses that affect the overall efficiency of DC machines. With this knowledge, engineers can develop strategies to improve energy use and performance.
Efficiency redefined
The idle loss method redefines efficiency assessment by providing insight into the machine performance scenario beyond traditional test scenarios. It highlights losses that are often not in the spotlight and highlights their importance in the overall efficiency equation.
Determination of the thermal resistance of the windings
Temperature and resistance
Winding hot resistance refers to the resistance of a winding operating under load and at elevated temperature. Unlike cold resistance, which is measured at room temperature, heat resistance reflects the resistance when the winding is heated due to current flow and other factors.
Operation under load
To determine heat resistance, the winding is subjected to a load that corresponds to its typical operating conditions. As current flows through the winding, it generates heat due to the inherent resistance of the winding material.
Measuring change
The main aspect of this measurement is to track the change in resistance as the winding heats up. This is critical because most materials, including the copper used in windings, experience an increase in resistance as the temperature increases. The increase in resistance is proportional to the increase in temperature.
Meaning and applications
Determining heat resistance has critical implications in several areas, from energy generation to industrial processes. It provides information about winding performance under operating conditions, allowing engineers to optimize designs and optimize energy use.
Challenges and calibration
Accurately measuring heat resistance can be challenging due to factors such as the temperature distribution within the winding and the influence of other materials. To ensure accurate results, special measuring techniques and devices are used.
Improving efficiency and reliability
By understanding the behavior of winding resistance under operating conditions, engineers can make informed decisions about system design, resiliency and component life. This knowledge contributes to the overall efficiency and reliability of electrical systems.
A precision instrument
Determining the thermal resistance of windings is a precision tool in the engineer's toolbox. Enables a deeper understanding of the performance of electrical components in real-world scenarios, improving the accuracy and effectiveness of design and operation.
Limitations of Testing and Accuracy Issues
Determination of constant losses
How to determine efficiency when operating as an engine:
To determine efficiency when operating as a generator:
Advantages and disadvantages
Per
-
Since this test is a stress test, less energy is required. Therefore the cost is economical.
-
The efficiency of the machine can be determined easily because the constant losses are known.
-
This test is appropriate.
Disadvantages
-
When charging the DC machine, the parasitic load losses that occur are not taken into account in this test.
-
With this method we cannot check the performance of the DC machine at full load.
Conclusion
At the end of this investigation, we realized that electrical engineering is not just about energy use; it’s also about understanding them. It's about unlocking the secrets of resistance, losses and performance under different conditions. It's about using this knowledge to redesign the way we design, operate and manage electrical systems.