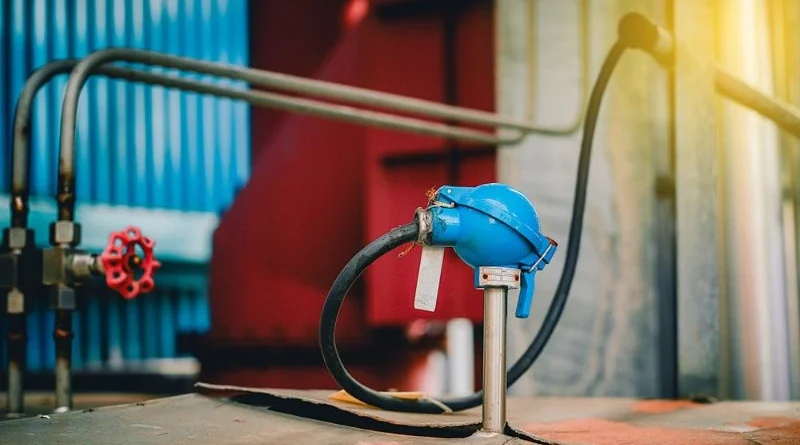
Os detectores de temperatura de resistência (RTDs) são soluções incrivelmente precisas e estáveis para medição de temperatura. Eles estão continuamente transformando indústrias, desde o processamento de alimentos até o aeroespacial. Você já se perguntou como funciona essa inovação? Mergulhe conosco no mundo complexo, mas fascinante, dos RTDs! Vamos descobrir como eles funcionam, explorar por que se tornaram um pilar da medição de temperatura e explorar como eles podem revolucionar suas operações. Esteja preparado para redefinir o que você sabe sobre termodinâmica enquanto desmistificamos o funcionamento dos RTDs.
O princípio de funcionamento de um RTD baseia-se na propriedade da resistência elétrica, que muda com a temperatura. Os RTDs utilizam metais como platina, cobre ou níquel, cuja resistência aumenta ou diminui à medida que a temperatura diminui. Esta mudança na resistência é medida e usada para determinar com precisão a temperatura. MyTech-Info tem um artigo que explica detalhadamente o princípio de funcionamento dos RTDs e contém informações sobre várias configurações e métodos de conversão de sinal.
Compreendendo a tecnologia RTD
Uma das tecnologias mais comumente usadas para medição precisa de temperatura é o detector resistivo de temperatura (RTD). Para entender como funciona, precisamos entender seus princípios subjacentes. Basicamente, um RTD baseia-se na suposição de que a resistência elétrica dos metais muda com a temperatura. Este princípio baseia-se no facto de que à medida que a temperatura aumenta, a resistência eléctrica de certos metais como a platina, o cobre e o níquel também aumenta. Da mesma forma, sua resistência diminui à medida que a temperatura diminui.
Os detectores de temperatura resistivos podem fornecer medições de temperatura confiáveis e de alta precisão em diversas aplicações. Eles são frequentemente usados em processos industriais onde a precisão é crítica, como: B. em fábricas, refinarias e laboratórios científicos. Ao compreender como funcionam os RTDs, podemos ver a sua importância em diferentes indústrias.
Platina, cobre e níquel em RTDs
Entre os metais utilizados em RTDs, a platina é popular devido à sua excelente estabilidade e resposta linear às mudanças de temperatura. Ele exibe desvio mínimo ao longo do tempo e fornece alta precisão em uma ampla faixa de temperatura. Essas propriedades desejáveis tornam os RTDs de platina adequados para aplicações exigentes onde a precisão é de extrema importância.
Cobre e níquel também são usados em RTDs, mas são menos comuns que a platina. O cobre tem uma faixa de temperatura mais ampla que a platina, mas pode ser suscetível à corrosão em ambientes agressivos. O níquel, por outro lado, oferece alternativas econômicas para aplicações em temperaturas mais baixas, mas pode resultar em erros de medição maiores.
Cada metal contribui com suas propriedades exclusivas para o desempenho de um sensor RTD. A escolha do material depende de fatores como considerações de custo, faixa de temperatura desejada, requisitos de estabilidade e condições ambientais da aplicação.
Por exemplo, um RTD à base de platina seria mais adequado para um ambiente industrial com temperaturas extremas ou materiais corrosivos devido à sua robustez e precisão. Em contraste, um RTD de cobre ou níquel poderia ser escolhido para aplicações menos críticas, onde a eficiência de custos é priorizada em detrimento da precisão máxima.
Compreender as vantagens e limitações desses metais em RTDs é fundamental para selecionar o sensor certo para necessidades específicas de medição de temperatura. É sempre recomendável consultar especialistas ou consultar as especificações técnicas dos fabricantes para tomar decisões informadas.
Configurações comuns de RTD
Quando se trata de RTDs, existem algumas configurações comumente usadas que atendem a diferentes necessidades de medição de temperatura. As mais populares incluem as configurações de 2 fios, 3 fios e 4 fios.
Na configuração de 2 fios, o RTD é conectado em série com o medidor, resultando em imprecisão devido à resistência do condutor. Esta configuração é normalmente usada para aplicações de curta distância onde a alta precisão não é crítica.
A configuração de 3 fios resolve o problema da resistência da linha introduzindo um fio adicional que a compensa. Esta configuração oferece maior precisão e é amplamente utilizada em diversas aplicações industriais.
A configuração de 4 fios melhora ainda mais a precisão usando dois condutores adicionais, criando um verdadeiro circuito de ponte que elimina os efeitos da resistência dos condutores na medição. Consequentemente, esta configuração oferece o mais alto nível de precisão e é frequentemente utilizada em medições de temperatura exigentes e precisas.
A configuração apropriada do RTD depende da precisão necessária, das limitações da aplicação e do custo. A compreensão dessas configurações comuns permite tomar decisões informadas ao configurar sistemas de medição de temperatura.
- De acordo com o Instituto de Engenheiros Elétricos e Eletrônicos, os RTDs à base de platina podem atingir uma precisão de até 0,1°C.
- De acordo com a Sociedade Americana de Testes e Materiais, a configuração de 3 fios é a configuração de RTD mais comumente usada em ambientes industriais, representando aproximadamente 60% das aplicações de RTD.
- De acordo com um relatório da Allied Market Research, o mercado global de IDT foi avaliado em cerca de 1,4 mil milhões de dólares em 2020, com uma CAGR estimada de 6,2% de 2021 a 2028, indicando a sua utilização generalizada em processos de medição de temperatura em todo o mundo.
Princípio de funcionamento da IDT
Para compreender como funciona uma IDT, precisamos de examinar o seu princípio de funcionamento. Os RTDs funcionam com base no conceito fundamental de que a resistência elétrica muda com as flutuações de temperatura. Em outras palavras, à medida que a temperatura aumenta, a resistência de certos metais utilizados em sensores RTD, como platina, cobre ou níquel, também aumenta.
Esta relação entre temperatura e resistência pode ser descrita matematicamente usando uma equação básica conhecida como equação de Callendar-Van Dusen: RT = Rref (1 + α (T – Tref)). Aqui, TR representa a resistência a uma determinada temperatura (T), referência denota a resistência de referência a uma temperatura de referência (reunião), E α denota o coeficiente de resistência da temperatura.
No entanto, é importante observar que podem ocorrer discrepâncias ao comparar a resistência calculada com as tabelas RTD. As características não lineares dos RTDs e as variações nos processos de fabricação podem contribuir para essas variações, especialmente em temperaturas Fahrenheit.
Embora a equação de Callendar-Van Dusen forneça uma estimativa de temperatura útil com base na resistência, ela nem sempre resulta em uma correspondência exata. Portanto, o uso de tabelas RTD ou dispositivos de calibração é recomendado para medições precisas de temperatura.
Por exemplo, imagine um processo industrial onde a temperatura precisa ser monitorada dentro de tolerâncias rígidas. Uma compreensão precisa da interação entre resistência e temperatura é crucial neste contexto. Ao utilizar RTDs e os princípios acima, os operadores podem garantir que os processos sensíveis funcionem de forma otimizada.
Interação entre resistência e temperatura
Compreender a interação entre resistência e temperatura é fundamental para entender como funcionam os RTDs. Conforme mencionado anteriormente, os RTDs baseiam-se no fato de que a resistência elétrica dos metais muda com a temperatura. Mais especificamente, a resistência dos metais aumenta à medida que a temperatura aumenta e diminui à medida que a temperatura diminui. Esta relação é descrita por uma equação matemática, a equação de Callendar-Van Dusen ou uma versão simplificada, a equação Alpha.
Um material comumente usado em sensores RTD é a platina porque possui uma relação resistência-temperatura estável e previsível. No entanto, é importante notar que a mudança na resistência não é linear e pode variar dependendo da difusão das impurezas e dos fatores de brilho. Além disso, diferentes fabricantes podem utilizar platina de pureza diferente, resultando em diferentes coeficientes alfa. Portanto, podem ocorrer discrepâncias ao comparar medições de RTD com tabelas padrão ou ao usar equações específicas.
Considere este cenário: Você tem um sensor RTD de platina e mede sua resistência em duas temperaturas diferentes com um multímetro. De acordo com a equação alfa, espera-se uma certa mudança na resistência com base na diferença de temperatura entre esses dois pontos. Entretanto, quando você compara suas medições com uma tabela RTD, elas não concordam perfeitamente. Essa discrepância pode ser atribuída a diversos fatores, incluindo incertezas nos processos de fabricação e variáveis que afetam as propriedades do metal.
Também vale a pena mencionar que a maioria das aplicações industriais utiliza configurações de três fios para seus RTDs porque podem minimizar os efeitos da resistência da linha. O circuito da ponte de Wheatstone é fundamental para converter essas mudanças de resistência em sinais mensuráveis de tensão ou corrente. Transmissores de temperatura ou dispositivos como módulos PLC, controladores DCS ou PID são geralmente usados para processar esses sinais e fornecer valores de temperatura significativos.
Projeto prático de circuito RTD
Ao projetar circuitos práticos para RTDs, devem ser feitas considerações para garantir uma medição precisa da temperatura. A escolha da configuração do RTD, seja de dois fios, três fios ou quatro fios, depende dos requisitos específicos da aplicação. As configurações de três fios são frequentemente preferidas em ambientes industriais porque compensam os efeitos da resistência da linha.
O circuito da ponte de Wheatstone é usado para medir com precisão uma mudança na resistência. Este arranjo de ponte permite que pequenas alterações na resistência sejam detectadas, equilibrando uma tensão de excitação nas pernas da ponte. A diferença de tensão entre certos pontos indica a extensão da mudança na resistência e, portanto, a temperatura correspondente.
A implementação de uma fonte de tensão de excitação apropriada, valores precisos de resistência da ponte e técnicas cuidadosas de fiação são fatores essenciais que contribuem para medições confiáveis e estáveis. Além disso, pode ser necessária blindagem para minimizar ruídos e interferências que comprometam a integridade do sinal.
Agora que desenvolvemos uma compreensão da interação entre resistência e temperatura e examinamos considerações práticas de projeto de circuitos para RTDs, podemos nos aprofundar em outro aspecto crucial: o papel do circuito da ponte de Wheatstone em permitir medições precisas de temperatura.
Papel da Ponte Wheatstone
Para compreender como funcionam os RTDs, é importante compreender o papel de um componente-chave denominado ponte de Wheatstone. A ponte de Wheatstone é um circuito elétrico especial que desempenha um papel importante na conversão das alterações na resistência de um sensor RTD em uma tensão ou corrente mensurável. Ao usar dois conjuntos de resistores em uma configuração balanceada, qualquer alteração na resistência pode ser detectada com precisão e convertida em um sinal elétrico proporcional.
Pense na Ponte Wheatstone como uma balança com dois braços. O resistor de referência fixo (Rref) está localizado em um braço; por outro lado, a resistência do sensor RTD variável (RT). Quando o calor afeta a resistência do sensor RTD e a altera, a ponte fica desequilibrada. A tensão ou corrente de saída produzida por este desequilíbrio é então medida e interpretada para determinar a temperatura correspondente.
O circuito ponte de Wheatstone é um importante intermediário entre o sensor RTD e os dispositivos de medição de temperatura, como transmissores de temperatura ou módulos PLC, permitindo medições de temperatura precisas e confiáveis.
Agora que entendemos o papel da ponte de Wheatstone nas medições de RTD, vamos examinar por que os RTDs são a escolha preferida para medições de temperatura e quais vantagens eles oferecem.
- A Ponte de Wheatstone é uma parte crucial para a compreensão de como funcionam os RTDs. Ele converte as alterações na resistência em um sensor RTD em uma tensão ou corrente mensurável. Ao usar dois conjuntos de resistores em uma configuração balanceada, qualquer alteração na resistência pode ser detectada com precisão e convertida em um sinal elétrico. A ponte Wheatstone atua como intermediária entre o sensor RTD e os dispositivos de medição de temperatura, permitindo a determinação de leituras de temperatura precisas e confiáveis. Esse conhecimento nos ajuda a entender por que os RTDs são a escolha preferida para medição de temperatura e quais vantagens eles oferecem.
Por que escolher RTDs? Vantagens explicadas
Quando se trata de medições de temperatura precisas e precisas, os RTDs oferecem diversas vantagens sobre métodos alternativos, como termopares. Uma vantagem importante é sua maior precisão devido às suas características de resistência estáveis e repetíveis. Os RTDs fornecem leituras confiáveis e consistentes, tornando-os ideais para aplicações onde a precisão é necessária.
Outra vantagem significativa é a sua maior linearidade em comparação com outros sensores de temperatura. A mudança na resistência em um RTD segue uma relação mais previsível e linear com as flutuações de temperatura. Isto facilita a calibração e a compensação de desvios, garantindo medições de temperatura mais precisas em uma ampla faixa.
Em contraste com outros sensores de temperatura, os RTDs apresentam excelente estabilidade a longo prazo. Os materiais comumente usados em elementos RTD, como platina, cobre ou níquel, apresentam desvio mínimo ao longo do tempo. Essa estabilidade ajuda a manter a precisão das medições de temperatura por longos períodos de tempo, tornando os RTDs uma escolha confiável para processos industriais que exigem monitoramento contínuo.
Além disso, os RTDs são menos suscetíveis a interferências eletromagnéticas devido à sua baixa impedância de saída. Isso os torna adequados para ambientes com alto ruído elétrico ou próximos a outros dispositivos eletrônicos, sem causar degradação ou imprecisão do sinal.
Além disso, a disponibilidade de diferentes configurações de RTD (2 fios, 3 fios e 4 fios) permite que os usuários escolham a opção mais adequada com base nos requisitos específicos de sua aplicação. Por exemplo, a configuração de 3 fios é frequentemente usada em ambientes industriais para equilibrar a resistência dos fios condutores e melhorar a precisão da medição.
Escolher um RTD para medição de temperatura é como escolher uma bússola confiável para navegar em terrenos difíceis – ele fornece orientação precisa e confiável.