Weldability refers to the ability of a material to be welded into components that meet specified design requirements under defined construction conditions and that satisfy predetermined service requirements.
Weldability is influenced by four factors: material, welding method, component type and usage requirements.
I. Iron-Carbon Alloy
1 . Overview
The Iron-Carbon alloy is a binary alloy composed of iron and carbon. It is the most used type of iron-based material. Carbon steel and cast iron are iron-carbon alloy materials. Alloys with less than 0.0218% carbon are known as industrial pure iron. Iron-carbon alloys with less than 2.11% carbon are called steel.
Alloys with more than 2.11% carbon are known as cast iron. In addition to carbon, carbon steel and cast iron contain impurities such as silicon, manganese, sulfur, phosphorus, nitrogen, hydrogen and oxygen; these impurities can affect the properties of the steel.
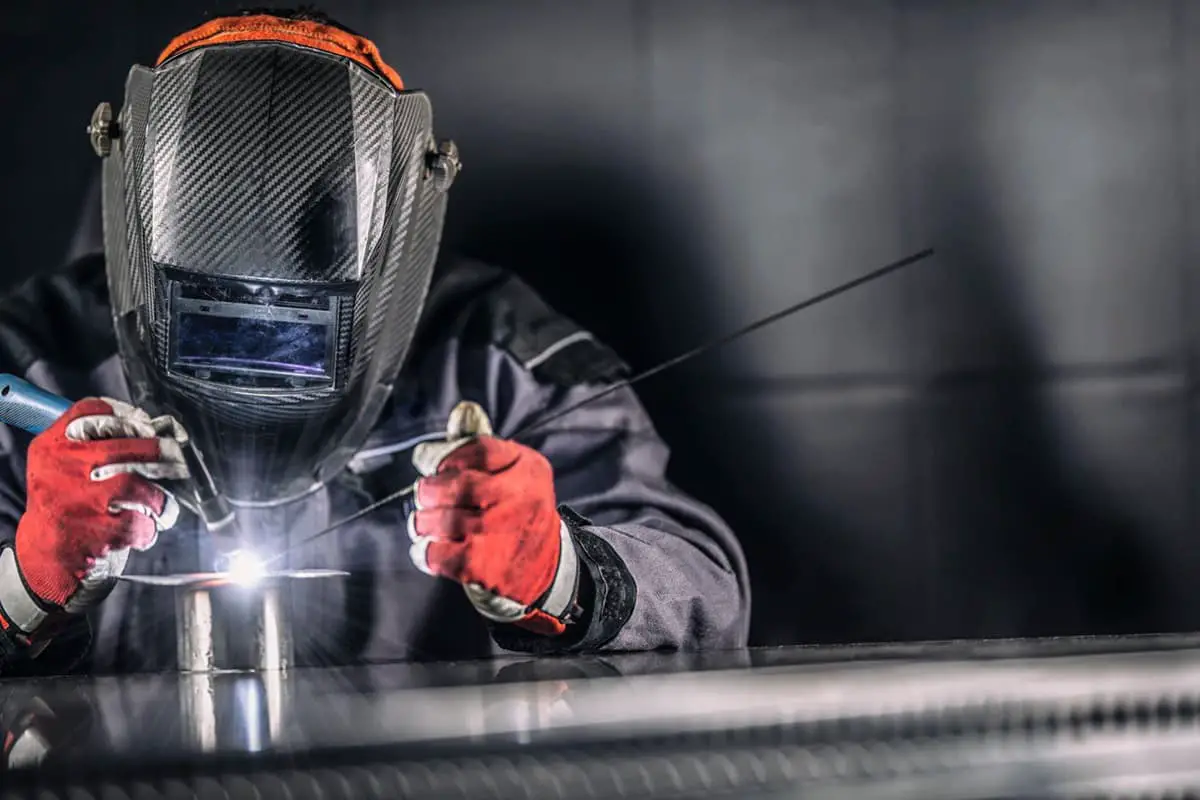
Table 2-1: Weldability of Various Types of Carbon Steel
Name | carbon content | Typical Hardness | Typical uses | Weldability |
Low carbon steel | ≤0.15% | HRB | Special sheets and thin profiled sheets, strips and welding wires | Great |
0.15%~0.25% | 30-35HRB | Structural profiles, plates and bars | Good | |
Medium Carbon Steel | 0.25%~0.60% | 42-46HRC | Mechanical parts and tools | Medium (typically requires preheating, low hydrogen welding method recommended) |
High carbon steel | >0.60%-1.00 | 55HRC | Springs, molds | Weak (requires low hydrogen welding method, preheating and postheating) |
Although the strength of carbon steel is relatively low, it is easy to cast, has excellent processability and is inexpensive. It has superior forgeability, weldability and cutting performance. Carbon steel is typically supplied to the market in various shapes and sizes, such as round steel, square steel, I-beam steel, and rebar.
Low carbon steel, with carbon content as low as 0.25% or less, and minimal M and Si content, has a low tendency to harden, making it the most weldable type of steel. In addition to C, M and Si, impurities such as S, P, O, N in carbon steel can affect its mechanical properties, cold cracking of welded joints, hot cracking and sensitivity to aging brittleness. The mechanical properties of common low-carbon steel are shown in Table 2-2.
Table 2-2 Mechanical Properties of Low Carbon Steel
Note | Level | Tensile test (not inferior) | Impact test | Cold bending test 180°, B=2a |
||||
Yield point /MPa |
Tensile strength /MPa |
Elongation Rate | Temperature /℃ |
Charpy V-Notch Impact Absorption Energy (Longitudinal) /J≥ | ||||
Bending Core Diameter d |
||||||||
Longitudinal Specimen | Transverse Specimen | |||||||
Q195 | – | 195 | 315-430 | 33 | – | – | 0 | 0.5a |
Q215 | A | 215 | 335-410 | 31 | – | – | 0.5a | The |
B | 20 | 27 | ||||||
Q235 | A | 235 | 370-500 | 26 | – | – | The | 1.5a |
B | 20 | 27 | ||||||
– | 0 | |||||||
D | -20 | |||||||
Q275 | A | 275 | 410-540 | 22 | – | – | 1.5a | 2a |
B | 20 | 27 | ||||||
W | 0 | |||||||
D | -20 | |||||||
Q245R | – | 245 | 400-520 | 25 | 0 | 31 | 1.5a | |
(20g,20R) |
Forms:
- Q195, Q215 and Q235 have good plasticity and can be rolled into steel plates, rebars, steel pipes and more.
- Q255 and Q275 can be rolled into structural steel, steel plates, etc.
Generally, no heat treatment is carried out after forming. Most of them are used directly in the hot-rolled state.
2. Weldability of Carbon Steel
Carbon steel and low-alloy steel are categorized based on their chemical compositions into three groups: carbon steel, low-alloy steel and alloy steel, with carbon steel (commonly referred to as carbon steel) being the most widely used.
In addition to carbon, which is the main alloying element of carbon steel, there is also silicon (Si<0.5%), manganese (Mn<0.8%) and inevitable impurities such as sulfur and phosphorus.
Low alloy steel is derived from carbon steel and one or more alloying elements are intentionally added to obtain certain properties. For example, 16Mn steel.
Classification and use of alloy steel:
- Structural Alloy Steel
- Alloy Tool Steel
- Special Performance Steel
Total alloying element content:
- Low alloy steel: <5%
- Medium alloy steel: 5% – 10%
- High alloy steel: >10%
1. Weldability analysis of low carbon steel
The weldability of carbon steel deteriorates with increasing carbon content. When selecting welding materials, not only must they match the original material in composition and properties, but harmful elements such as sulfur and phosphorus in the weld metal must also be avoided.
When welding carbon steel with a carbon content greater than 0.25%, the source of hydrogen must be minimized.
The mechanical reasons for cracking during welding of carbon steel are structural restraint stresses and uneven thermal stresses. Different technological measures will have to be taken depending on the carbon content.
For low-carbon steel, special attention must be paid to avoid cracks caused by structural restraint stresses and uneven thermal stresses. In addition to preventing cracks caused by these stresses, steel with a high carbon content must mainly prevent cracks caused by hardening.
The weldability of carbon steel depends mainly on its susceptibility to cold cracking, hot cracking and the toughness of the joint. The carbon content of the steel and deposited metal has the most significant impact on the cold cracking of carbon steel.
Carbon Equivalent: CE=C+Mn/6+Si/24
For carbon steel, the silicon content is relatively low, not exceeding 0.5%. Sometimes its impact can be ignored. As the Carbon Equivalent (CE) value increases, the propensity for cold cracking increases and weldability deteriorates. Typically, when the EC value exceeds 0.40%, the sensitivity to cold cracking increases.
The tendency for weld and heat-affected areas to harden, and their susceptibility to cold cracking, are not only related to the composition, but the impact of the structure on performance is even more significant. Given a given composition, the structure depends on the cooling rate, which can be determined through its SHCCT (Simulated Heat Affected Zone Continuous Cooling Transformation) structure. Figure 2-2 presents the SHCCT diagram of Q235 (A3) steel.
Controlling the cooling rate of the welding area is a crucial method for changing the type of structure and hardness of the welding zone, thus reducing the occurrence of cold cracks.
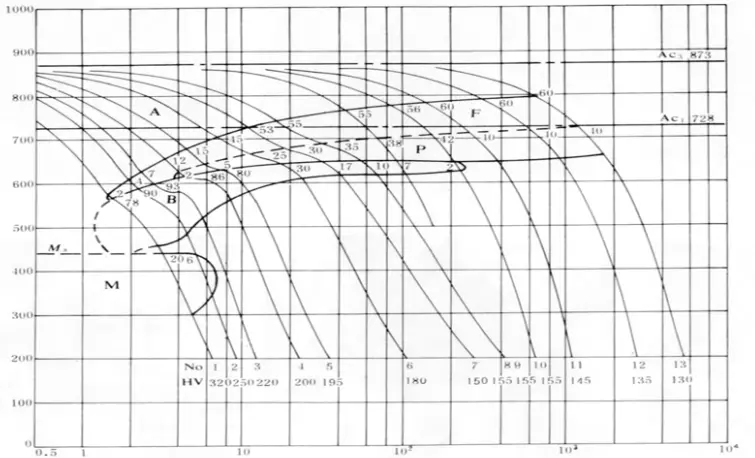
As the welding thickness increases, or when the ambient temperature is lower during welding, or the welding wire energy is lower, heat dissipation accelerates and the cooling speed of the welded joint increases, which in turn the tendency to cold cracks increases.
T-joints and lap joints have a larger heat dissipation area compared to butt joints, thus accelerating the cooling rate in the welding zone and making it prone to the formation of hardened structures.
Preheating, increasing the temperature between welding tracks or layers, or postheating measures can also reduce the cooling rate during welding.
The hardening of carbon steel is mainly due to the formation of martensitic structures. Martensite is a supersaturated solution of carbon in α-Fε, and its hardness is related to both the carbon content in the steel and the amount of martensite formed. The amount of martensite is influenced by the cooling rate; a very fast cooling speed can produce 100% martensite, thus achieving the highest hardness.
Hydrogen and Degree of Restriction
The hydrogen in the welding area mainly comes from the welding materials and moisture in the welding zone. Dissolved hydrogen in the weld can be reduced by using low-hydrogen welding materials, increasing the drying temperature of the welding materials, reducing the moisture content in the shielding gas, or decreasing the humidity in the welding area.
An increase in the thickness of the steel plate or the stiffness of the structure will increase the degree of restraint, thereby increasing the sensitivity to hydrogen-induced cracking.
The three main inducers of cold crack sensitivity are hardened structure, hydrogen and restraint stress. When the composition of the steel is fixed, the greater the proportion of the hardened structure, the lower the critical hydrogen content required to cause cold cracking and the lower the restraint stress required, thus increasing the tendency for cold cracking.
When the structure and hydrogen content are fixed, the greater the degree of restriction, the greater the sensitivity to cold cracking. Therefore, in the tendency of carbon steel to cold cracking, the three factors of hardened structure, hydrogen and restraint stress promote each other and are interdependent.
Hot Crack
Susceptibility to hot cracking is closely related to impurities such as sulfur (S) and phosphorus (P) in steel. During welding of carbon steel with high S and P content, low-melting point S and P compounds accumulated at the grain boundaries in the heat-affected zone, causing settlement cracks near the fusion line of the heat-affected zone. heat.
For thicker steel sheets, sulfides distributed along different segregation zones can lead to laminar cracks in T-joints and others. When the dilution rate of the base material is high, more S and P enter the weld seam, which can easily cause hot cracking of the weld seam.
To avoid this, the joint design or process operation must prevent the weld seam from having a narrow and deep shape. Low carbon steel arc welding beads generally have greater resistance to hot cracking.
laminar tear
Boiling steel has a higher oxygen content and a noticeable segregation band in the center of the plate thickness, which can cause cracking and porosity during welding. There is a certain tendency to laminar rupture when welding thick plates and it also presents a high degree of sensitivity to aging. The brittle transition temperature of the welded joint is also on the higher side.
Therefore, boiling steel is not typically used in the manufacture of structures subject to dynamic loads or operating at low temperatures.
Changes in heat affected zone performance during welding
The main change is in the ductility of the joint, which depends on the composition of the steel, the state of heat treatment of the base material before welding and the thermal welding process. Carbon steel is mainly delivered in hot-rolled state, but for some high-quality carbon structural steels and special-purpose carbon structural steels, the delivery state can also be controlled rolling, normalizing, normalizing + tempering or quenching + tempering .
During the steel cooling process, a large strain is applied at a lower temperature before the austenite decomposes, which increases the nucleation rate of the ferrite phase. The resulting grains are significantly refined, thus drastically improving their strength and ductility.
Certain welding methods have dispersed heat sources or excessively high linear energy, such as gas welding and electroslag welding. These methods cause the grains in the coarse grain zone of the heat-affected area of the weld to become even larger, thus reducing the impact strength of the joint. Consequently, post-weld heat treatment is often required for significant structures.
In conclusion, low carbon steel has low carbon content and very low alloying element content. Therefore, using conventional welding methods, there will be no hardened structures or cold cracks at the joints. As long as welding materials are selected correctly, satisfactory welding joints can be achieved.
2. Welding methods
There are no specific requirements for choosing a welding method for low carbon steel. Selection can be made based on variables such as material thickness, product structure, performance requirements and production conditions.
Shielded metal arc welding, CO 2 gas shielded welding, and submerged arc welding are common welding methods.
3. Weldability analysis of medium carbon steel
Cold crack
Medium carbon steel has a higher carbon equivalent than low carbon steel. When the carbon content exceeds 0.25%, the hardenability of steel increases. During welding, if the cooling speed is fast (as shown in the CCT diagram of 45 steel in Figure 2-3, the cooling speed exceeds the critical value point c), a martensitic structure will form in the heat-affected zone.
The martensitic structure of medium carbon steel is more brittle and, under the action of welding stresses, is subject to cold cracking and brittle fractures. The heat affected zone of medium carbon steel is more likely to form a hardened martensitic structure after welding. This structure is more sensitive to hydrogen and the critical stress required for cold cracking is lower.
Therefore, it is advisable to use electrodes with low hydrogen content and appropriately increase the preheating temperature to reduce residual voltage.
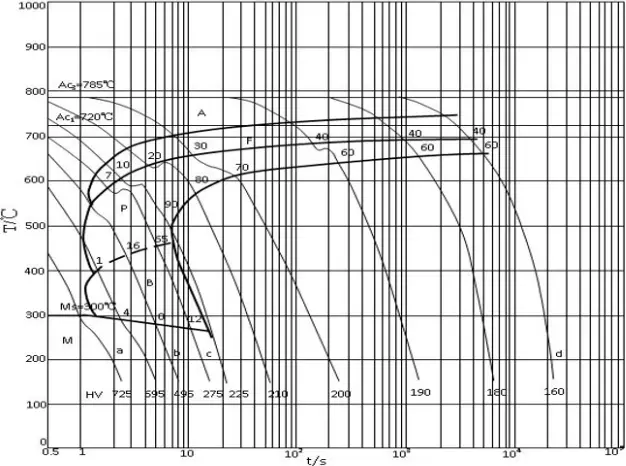
Hot Cracks
When the mass fraction of carbon in steel exceeds 0.25%, the segregation of carbon itself and its promotion from other elements such as sulfur (S) and phosphorus (P) become significantly prominent. This is especially the case when the S and P content in the original material is controlled close to the upper limit of the qualified value required by the standard. Low melting point sulfides can lead to an increased tendency for hot cracking, particularly in the arc well.
Therefore, when welding carbon steel, strict control of the sulfur mass fraction is essential.
Pores
Due to the higher carbon content compared to low carbon steel, the amount of carbon entering the weld pool increases. This could potentially lead to the formation of CO pores during metallurgical welding reactions, thereby increasing the sensitivity to pore formation in the weld seam.
When welding boiling steel, it is crucial to ensure that the chosen welding material contains suitable deoxidizers to prevent the formation of pores in the weld seam.
Changes in heat affected zone performance in welding
The zone affected by heat during welding undergoes changes due to the emergence of hardened structures, resulting in increased resistance, brittleness and hardening, as well as greater susceptibility to cold cracking. Carbon from the molten parent metal enters the weld pool, leading to an increase in the carbon content of the weld metal. Performance varies between weld paths due to differences in dilution rates.
As the carbon content in medium carbon steel increases, its weldability deteriorates. The main problems encountered during welding are hot cracking, cold cracking, porosity and brittle fracture, and sometimes there is a decrease in strength in the heat-affected zone. The more impurities in the steel and the greater the structural rigidity, the more serious these problems become.
When welding repair work is carried out on medium carbon steel castings, precautions must be taken to prevent cold cracking during welding or cracking due to excessive residual stress in the repaired area.
4. Welding methods
Typically, coated electrode welding or CO2 gas shielded welding methods are used. When adding wear or corrosion resistant surfaces to medium carbon steel, or when repairing larger worn surfaces to medium carbon steel, submerged arc welding can also be utilized.
5. Weldability analysis of high carbon steel
Poor weldability
High-carbon steel, which includes structural carbon steel, carbon steel castings, and carbon tool steel, contains more than 0.6% carbon. The weldability of these materials is quite low and welding can result in hard, brittle martensite with a high carbon content. They have a high tendency to harden and crack. Given their poor weldability and high hardness, these types of steel are typically used in components or parts that require high hardness and wear resistance, rather than in the creation of welded structures.
Welding methods: Shielded metal arc welding and gas welding are commonly used for repair welding.
II. Stainless steel
1. Overview
Stainless steel refers to a type of steel that does not rust easily in the atmosphere; It is a steel that is more resistant to corrosion in specific acid, alkaline and saline conditions. Due to its excellent corrosion resistance, formability and toughness over a wide temperature range, stainless steel is widely used in petrochemicals, nuclear power, light industry, textiles, food and household appliances.
1. Classification of Stainless Steel
(1) Austenitic Stainless Steel
Austenitic stainless steel is distinguished by its non-magnetic properties, good low temperature performance, formability and weldability.
(2) Ferritic Stainless Steel
Ferritic stainless steel is characterized by strong magnetism, easy formability, rust resistance and corrosion resistance.
(3) Martensitic Stainless Steel
Martensitic stainless steel is known for its high strength and hardness, although its corrosion resistance is slightly lower than that of austenitic and ferritic stainless steel.
(4) Duplex Stainless Steel
Duplex stainless steel has a high yield strength, resistance to pitting and stress corrosion cracking, and is easy to form and weld.
(5) Precipitation hardening stainless steel
Precipitation-hardened stainless steel has a chromium content of about 17% and, together with elements such as nickel and molybdenum, not only has sufficient stainless properties, but also exhibits corrosion resistance comparable to austenitic stainless steel.
2. Role of alloying elements
Iron: It is the basic metallic element of stainless steel.
Chromium: It is the main element that forms ferrite. When combined with oxygen, chromium forms a passive, corrosion-resistant Cr2O3 film, making it the essential element in maintaining the corrosion resistance of stainless steel.
Carbon: It is a strong austenite-forming element that can notably increase the strength of steel. However, carbon can also negatively affect corrosion resistance.
Nickel: It is the main element that forms austenite. Nickel can delay steel corrosion and grain enlargement during heating.
Molybdenum: It is an element that forms carbides. The carbides it forms are extremely stable, preventing grain growth during austenite heating and reducing the steel's sensitivity to overheating.
Niobium, Titanium: These are strong carbide-forming elements that increase the steel's resistance to intergranular corrosion.
Nitrogen: It is a strong austenite-forming element that significantly increases the strength of steel.
Phosphorus, Sulfur: These harmful elements of stainless steel negatively affect its corrosion resistance and stamping properties.
3. General physical properties of stainless steel
(1) Heat conduction: The heat transfer rate of stainless steel is relatively slow.
(2) Thermal Expansion: Compared with carbon steel, the linear expansion coefficient of grade 304 steel is higher.
(3) Electrical Resistance: Generally, the electrical resistance of alloys is higher than that of pure metals, and the same applies to stainless steel.
(4) Magnetic Properties of Stainless Steel
Table 3: Magnetic Properties of Various Materials
Materials | magnetic properties | Magnetic Permeability: μ(H=50e) |
SUS430 | Strong Magnetism | – |
Iron | Strong Magnetism | – |
No | Strong Magnetism | – |
SUS304 | Non-magnetic (exhibits magnetism during cold working) | 1.5(65% Processing) |
SUS301 | Non-magnetic (exhibits magnetism during cold working) | 14.8(55% Processing) |
SUS305 | Non-magnetic | – |
2. Weldability
1. Weldability of stainless steel
The electrical resistance of stainless steel is significantly greater than that of low carbon steel. During welding, both the welding rod and the base material in the welding area are prone to heat and melt. This can cause the surrounding base material to overheat, resulting in uneven deformation in the welding area and coarse grains.
Stainless steel has a high coefficient of linear expansion and a low coefficient of thermal conductivity, making heat dissipation difficult. During welding, the penetration depth is high and the welding heating causes the structure to expand. During cooling, significant shrinkage deformations and tensile stresses occur, which can easily lead to thermal cracking.
The heat affected zone (HAZ) in welding can easily lead to intergranular corrosion. This occurs because, within the HAZ, the base metal is depleted in chromium in the sensitization temperature range (450°C to 850°C), making passivation difficult.
As a result, its corrosion resistance decreases significantly and it is therefore preferentially corroded in the corresponding corrosive environment, widening the grain boundaries of the steel. At this point, the plasticity and strength of the corroded area have been severely compromised, leading to cracks and brittle fractures during cold bending and a non-metallic sound when the corroded location hits the ground.
Stainless steel is a type of steel that is relatively resistant to corrosion, but it is not completely rustproof. To date, no steel has been invented that does not corrode under any conditions. Therefore, specific types of steel are designed for use in certain environments.
The corrosion resistance of steel increases with the chromium content. When the chromium content reaches or exceeds 12%, the corrosion resistance of steel changes drastically, going from rust-prone to rust-resistant, and from non-corrosion-resistant to corrosion-resistant. Therefore, stainless steel is commonly referred to as an iron-based alloy with a chromium content greater than 12%.
III. Heat resistant steel
1. Overview
The ability of steel to maintain its chemical stability (resistance to corrosion and scale) at high temperatures is referred to as thermal stability; the property of steel to have sufficient strength at high temperatures is called thermal resistance. Steel that has thermal stability and heat resistance is known as heat-resistant steel.
1. Classification of Heat Resistant Steel
(1) In heat-resistant pearlitic steel, the primary alloying elements are chromium, molybdenum and vanadium, with their combined content normally less than 5%. This type is also known as low-alloy heat-resistant steel.
(2) Heat-resistant martensitic steel not only has high temperature resistance, but also has remarkable corrosion resistance. 1Cr13 and 2Cr13 steels can serve as heat-resistant steels as well as stainless steels.
(3) Heat Resistant Ferritic Steel
This type of steel has excellent resistance to high-temperature oxidation and corrosion, but has poor heat resistance and is prone to brittleness.
(4) Heat Resistant Austenitic Steel
This type of steel not only has high heat resistance, but also has considerable plasticity, toughness and excellent welding properties. Due to its single-phase austenitic structure, it also has superior corrosion resistance.
2. Weldability
1. Weldability of heat-resistant pearlitic steel
The main element of heat-resistant pearlitic steel is carbon and contains a certain amount of chromium and molybdenum. Some varieties also contain elements such as vanadium, tungsten, silicon, titanium and boron. The presence of these alloying elements makes the weld and the heat-affected zone prone to hardening.
After welding, air cooling can easily produce hard and brittle martensite, which not only affects the mechanical properties of the welded joint, but also generates significant internal stresses. Combined with a high concentration of hydrogen diffusion, the weld seam and heat-affected zone are prone to cold cracking.
Furthermore, because heat-resistant pearlitic steel contains strong carbides such as niobium, molybdenum and chromium, and is typically used at high temperatures, it is susceptible to cracking upon reheating.
2. Weldability of heat-resistant martensitic steel
Heat-resistant martensitic steel mainly includes high chromium steel with simple compositions such as Cr13 and 2Cr13, as well as steel that adds alloying elements such as Mo, V, W, Nb, etc., to the base of chromium 12. These Steel types tend to undergo air quenching, resulting in poor weldability. After welding, they generally form high hardness martensite and a small amount of bainitic structures, leading to cold cracking.
3. Weldability of heat-resistant ferritic steel
Most heat-resistant ferritic steel is composed of high chromium steel with w(Cr)>17% and a portion of Cr13 type steel. These types of steel do not undergo α→Y phase transformation during welding, and do not have a tendency to harden. However, the grains near the fusion line will increase rapidly, leading to brittleness of the welded joint.
The higher the chromium content and the longer the residence time at high temperatures, the more severe the brittleness becomes. This brittleness cannot be refined through heat treatment, making it prone to cracking when welding rigid structures.
4 . Weldability of heat-resistant austenitic steel
Heat-resistant austenitic steel has an austenitic microstructure as a matrix. This type of steel contains significant amounts of nickel, manganese and nitrogen, which are austenite-forming elements. It has excellent high temperature resistance and structural stability above 600℃, combined with good welding performance. Therefore, it is the most widely used type of heat-resistant steel in applications ranging from 600 to 1200 ℃.