Alkali-induced stress corrosion cracking, also known as “alkali embrittlement” or “alkali cracking,” has been documented in numerous literature sources. However, most research focuses on high temperature conditions, while there is relatively less research on the alkaline embrittlement of stainless steel at medium and low temperatures.
Although failures due to alkaline embrittlement can occur in high-temperature environments, such failures at medium and low temperatures are relatively uncommon.
In a domestic nuclear power plant, the hydrogen generator storage tank was made of 316L austenitic stainless steel and contained KOH solution, with a working pressure of 700-800 kPa. After eight years of service, the bottom of the tank developed cracks.
A thorough physical and chemical inspection and stress state analysis in different areas of the tank were carried out to determine the cause of the cracking. Based on the results, suggestions for improvements were proposed.
1. Physical and chemical inspection
1.1 Macroscopic observation and penetrant test
The pouring tank consists of a cylinder and an elliptical head, which are welded together. The head can be divided into a straight section and a curved section, as depicted in Figure 1a.
A through crack is present on the outer wall of the head and is located on the straight edge. The upper end of the crack is approximately 8 mm from the weld line, while the lower end is approximately 13 mm from the weld line. The total length of the crack is about 5 mm.
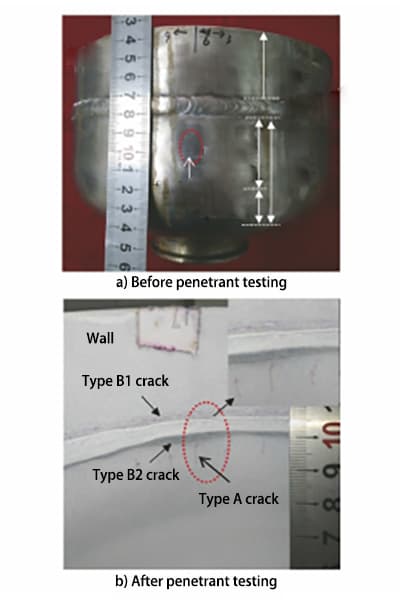
Liquid penetrant testing was performed on the tank and revealed that no additional cracks were present on the outer wall of the tank other than the through crack. However, multiple cracks were discovered on both sides of the inner wall near the weld. These cracks included axial cracks perpendicular to the weld and circumferential cracks parallel to the weld, as shown in Figure 1b.
Axial cracks were only present in the straight section of the head, 13 mm from the weld line. They had a uniform circumferential distribution and different lengths. The upper end of the longest crack was 1-2 mm from the weld line, while the lower end was approximately 13 mm away. The upper end of the shorter crack was approximately 4 mm from the weld line, with the lower end approximately 10 mm away. This crack was classified as a Type A crack, while the through crack was also classified as a Type A crack.
The circumferential cracks were located on both sides of the weld, 1-3 mm from the weld line. Cracks on the side of the cylinder were classified as Class B1 cracks, and cracks on the side of the cylinder head were classified as Class B2 cracks.
The macroscopic diagram of crack distribution in the leaking tank is shown in Figure 2.
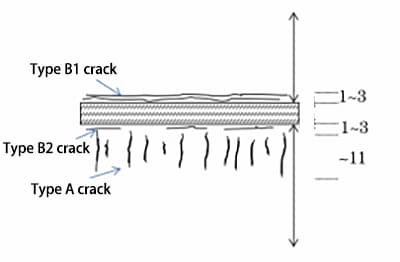
1.2 Analysis of chemical composition
The chemical composition of the cylinder and head base metal was analyzed using a spark direct reading spectrometer. The results showed that both chemical compositions meet the requirements specified in the ASTM A473-2017 standard.
1.3 Metallographic inspection
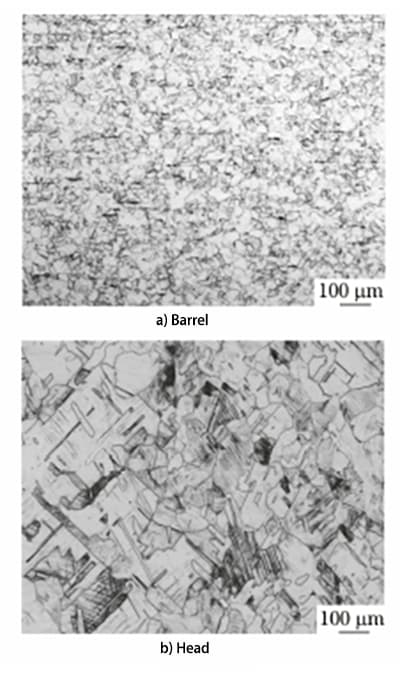
Samples were taken from the base metal of the barrel and head and subjected to metallographic inspection under an optical microscope. The microstructure of the tank's base metal is represented in Figure 3.
The microstructure of the base metal of the barrel was found to consist of austenite with a small amount of annealed twins and had a grain size of grade 6. Meanwhile, the microstructure of the base metal of the head was composed of austenite with a significant amount of strain twins and slip bands, and had a grain size of 3.5.
1.4 Hardness test
A Vickers digital hardness tester was used to measure the hardness of various parts of the tank. The results showed that the hardness of the barrel and base metal of the head was 165 HV and 248 HV, respectively.
The hardness of the weld, the heat-affected zone on the cylinder side, and the heat-affected zone on the head side were 171 HV, 188 HV, and 165 HV, respectively. The average thickness of the cylinder side and head was 3.71 mm and 4.24 mm, respectively.
The cylinder was made of 316L steel in the normal solution annealed state. The hardness of 316L steel is not specified in ASTM A473-2017. However, according to the standard of cold-rolled stainless steel plates and strips (GB/T 3280-2015), the hardness of 316L steel should not exceed 220 HV.
The high hardness of the head, which is related to the presence of a significant amount of deformation twins and slip bands in the structure, can be attributed to the fact that it is 316L steel in a cold-work hardened state.
1.5 Analysis of crack morphology
1.5.1 Crack surface analysis
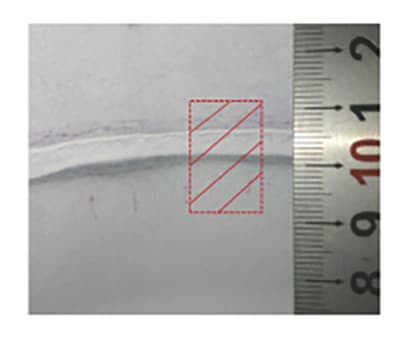
On the inner wall of the tank, Class A and Class B surface cracks should be sampled for analysis. The location of the sample is represented in Figure 4.
After the sample is flattened, polished and etched using an arc surface method, it is examined using an optical microscope. The microscopic morphology is illustrated in Figure 5.
It is apparent that both types of cracks extend toward the crystalline structure at the surface.
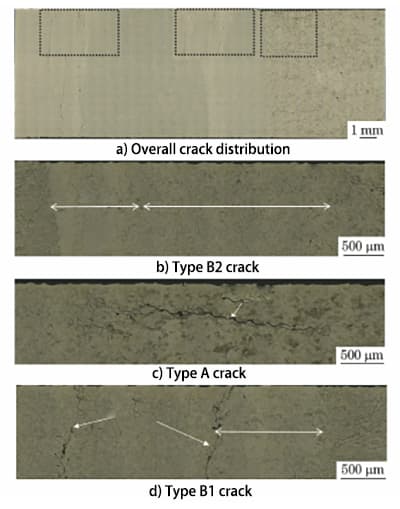
The central part of the Type A fissure is wide, while its two ends are narrow.
The heat-affected zone on the head side has a coarse-grained zone and a fine-grained zone, with a total length of approximately 4 mm.
The heat-affected zone on the cylinder side exhibits only a coarse-grained zone, approximately 0.8 mm in length, with no fine-grained zone present.
The base metal on the head side exhibits a significant number of deformation twins and slip bands, characterized by high deformation and distortion. Static recrystallization occurs during the welding process due to heating.
As a result of the high temperature near the weld, grain growth occurs after recrystallization and forms an area of coarse grains.
Only static recrystallization occurs in the area away from the weld, and the grain does not grow, resulting in a fine-grained area.
The base metal on the cylinder side is in a solution annealed state, with limited deformation and distortion and insufficient recrystallization driving force.
Due to the high temperature close to the weld, grain growth occurs directly, forming a coarse grain zone.
Since the temperature in the area away from the weld is lower than the grain growth temperature, only recovery occurs without recrystallization, and there is no fine grain area similar to that on the head side. As a result, it is not possible to directly determine the extent of the heat-affected zone.
The barrel and head materials are made of 316L stainless steel, with similar thermal conductivity. The extent of the heat affected zones on both sides of the weld is approximately the same.
Based on the extent of the heat-affected zone on the head side, it is inferred that the width of the heat-affected zone on the barrel is approximately 4 mm.
It is observed that one end of some Type A cracks is located in the heat-affected zone, while the other end is located in the straight section of the head, with the center located in the straight section of the head.
The remaining part of Type A fissures is located on the straight edge of the head.
All Type B cracks are located in the heat-affected zones on both sides of the weld.
1.5.2 Crack section analysis
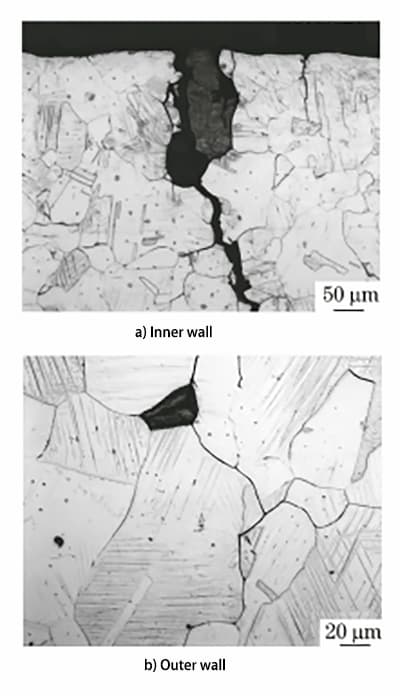
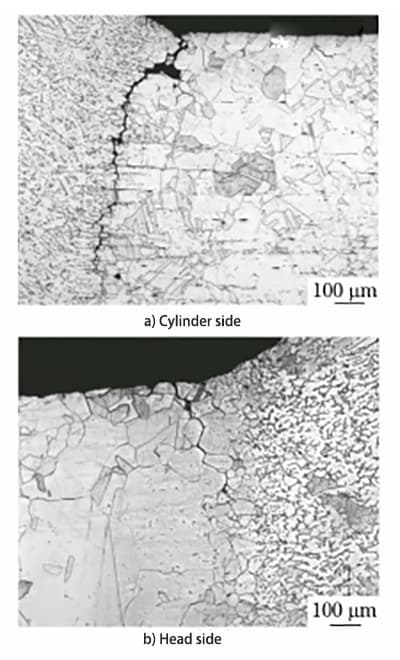
Figures 6 and 7 show the microstructures of the two types of cracks in the direction of the wall thickness.
Type A cracks run from the inner wall to the outer wall of the tank, following the crystal, with varying depths. The severe parts of these cracks cover almost the entire thickness of the tank wall, with a bifurcated crack tip and grain contour that is not sensitized. They have typical characteristics of corrosion cracking under intergranular stress.
Type B1 and B2 cracks are mainly found in the heat-affected zones on both sides of the weld. These cracks extend along the grain, with a bifurcated crack tip and a non-sensitized grain boundary, presenting typical characteristics of corrosion cracking under intergranular stress.
The microhardness of Type A, B1 and B2 cracks is 242 HV, 171 HV and 157 HV, respectively.
The sharp drop in hardness in the type B2 crack zone is due to the occurrence of static recrystallization after the welding process, which transforms the original deformed austenite grains.
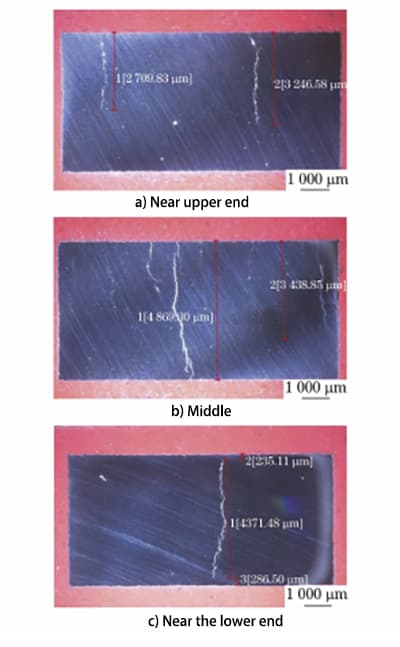
To obtain more information about the origin position of the Type A crack on the inner wall of the tank, the depth of the crack was dissected and measured in the center and on both sides along its longitudinal direction. The results are presented in Figure 8.
The deepest part of the crack along the wall thickness direction is found in the middle, indicating that the origin of the Type A crack is in the center of the length direction and extends from the inner surface of the wall toward both sides.
1.6 Residual stress analysis
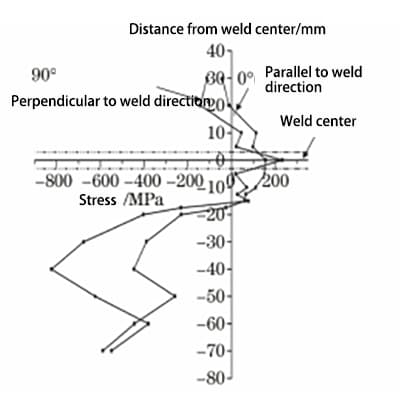
The Residual Stress Analyzer is used to evaluate the residual stress of the cylinder and head, with the weld serving as the limit. The test is performed in two directions, 0° (parallel to the weld direction) and 90° (perpendicular to the weld direction), and the results are shown in Figure 9.
The tensile residual stress zones on the cylinder side at 0° and 90° are approximately 20 mm and 12 mm, respectively, from the weld centerline. On the head side, the tensile residual stress zones in the 0° and 90° directions are approximately 17 mm and 15 mm away from the weld centerline, respectively.
Type A and Type B cracks are located within the residual tensile stress zones.
2. Comprehensive analysis
Type A and Type B cracks are found in the residual tensile stress zone of the tank, both running through the wall thickness, from the inner wall to the outer wall, in the direction of the crystal.
Type A cracks begin in the base metal area of the head and spread along the surface perpendicular to the weld on both sides.
Type B cracks are located in the heat-affected zone on both sides of the weld and extend parallel to the weld along the surface.
The head is manufactured using a cold stamping process.
The straight edge of the head is formed by flanging the original sheet edge inward, which results in significant plastic deformation and residual tensile stress.
Under the prolonged influence of the initial residual stress of cold working, a temperature of 65-70°C and the service conditions of an alkaline KOH solution, intergranular stress corrosion cracks are generated perpendicular to the weld.
The original residual stress disappears due to the recovery and recrystallization of austenite grains after welding in the heat-affected zone.
Welding residual tensile stress is produced in the heat-affected zone due to cooling contraction of austenite grains. This stress is mainly perpendicular to the weld, and under the prolonged effect of KOH alkaline solution service conditions at 65-70 ℃, corrosion cracking is generated under intergranular stress parallel to the weld.
The tank cracking mechanism can be explained by the membrane cracking theory of alkali-induced stress corrosion cracking.
In the alkaline KOH solution environment, a passive film forms on the surface of the inner wall of the tank, but it breaks under the high residual tensile stress.
Once the passivation film is broken, it does not immediately reform on the metal surface in the fracture area.
The bare metal then comes into contact with the KOH caustic soda, and the OH- concentrates in the fractured area of the surface, leading to a reaction with the bare metal.
The pure metal reacts with the concentrated lye to form a metal oxide film, but this film breaks again under stress, repeating the passivation-fracture cycle, causing the crack to continue to expand and extend. Eventually, the tank cracks and leaks.
3. Conclusions and suggestions
(1) The circumferential and axial cracks on the inner wall of the storage tank are caused by alkali-induced stress corrosion cracking.
Circumferential cracks are mainly caused by residual tensile stress from welding, while axial cracks are mainly due to residual tensile stress from cold working on the straight edge of the head.
(2) To avoid circumferential cracks, it is important to control the welding heat input and reduce the welding residual stress.
To avoid axial cracking, a stress relieving process must be added after cold forming of the head to reduce the residual stress from cold working.
(3) To ensure the safe operation of equipment, non-destructive testing measures such as penetration testing must be reinforced during operation for additional protection.