Almost half of the world's energy consumption is attributed to electric motors, so improving their efficiency is considered the most effective measure in addressing global energy issues.
Types of engines
Generally, motors convert the force generated by current flow in a magnetic field into rotational motion, but in general terms, this also includes linear motion.
Based on the type of power source that drives the motor, they can be classified into DC and AC motors.
In addition, according to the engine rotation principle, they can be roughly divided into the following categories, excluding special engines.
1. DC (direct current) motors
(1) Brushed motors
Widely used brushed motors are generally called DC motors. They rotate by sequentially contacting electrodes called “brushes” (on the stator side) and the “commutator” (on the armature side) to switch the current.
(2) Brushless DC Motors
Brushless DC motors do not require brushes and commutators. They use switching functions like transistors to switch current and achieve rotational motion.
(3) Stepper Motors
This motor operates in synchronization with the pulse power and hence it is also known as pulse motor. Its characteristic is the ability to easily achieve accurate positioning operation.
2. AC (Alternating Current) Motors
(1) Asynchronous Motors
AC current generates a rotating magnetic field in the stator, causing the rotor to induce current and rotate under its interaction.
(2) Synchronous Motors
The AC current creates a rotating magnetic field and the rotor with magnetic poles rotates due to the attraction. The rotation speed is synchronized with the power frequency.
stepper motor | Brushed DC Motor | Brushless DC Motor |
![]() |
![]() |
![]() |
Current, magnetic fields and force
Firstly, to facilitate subsequent explanations of motor principles, let's revisit the basic laws/rules relating to current, magnetic fields and force.
Although it may evoke a sense of nostalgia, this knowledge can be easily forgotten if magnetic components are not used regularly.
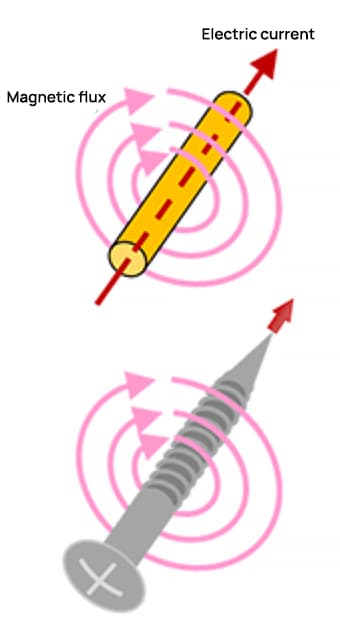
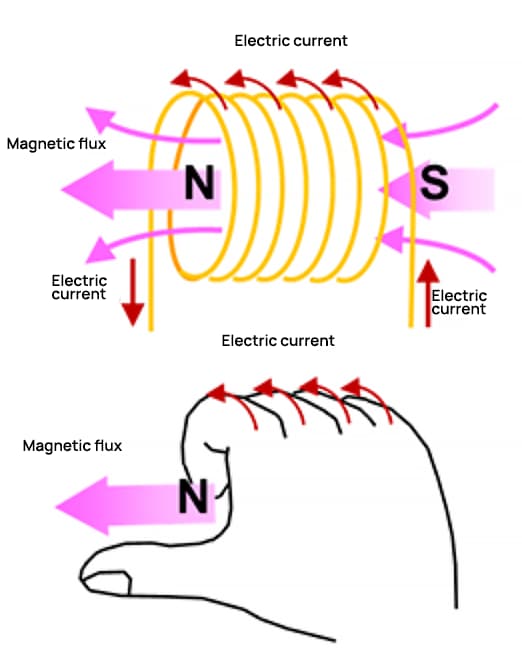
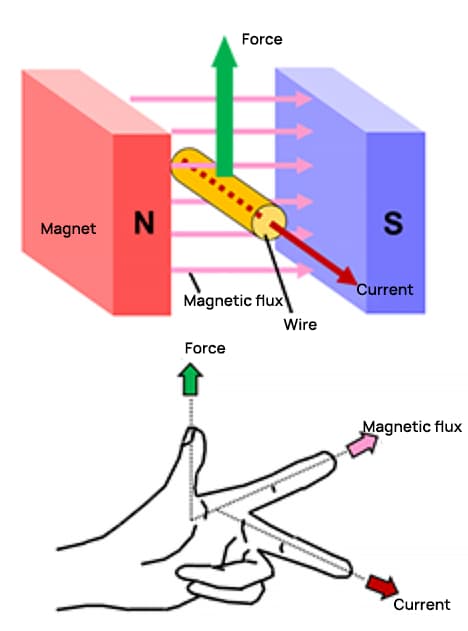
We will illustrate this using images and equations.
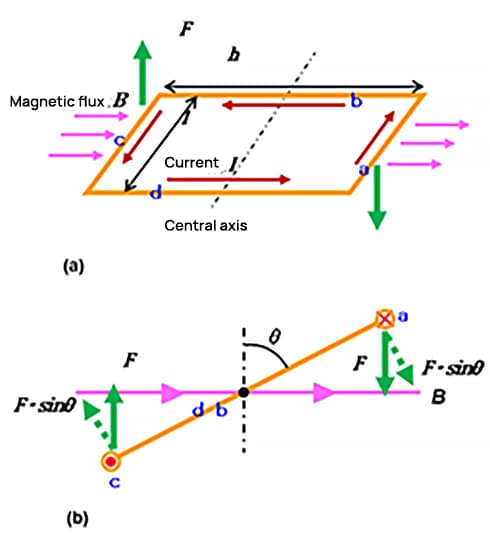
When the wire frame is rectangular, the force acting on the chain must be considered. The force F exerted on parts a and c of the structure is:

Torque is generated around the central axis. For example, when considering a state where the angle of rotation is just θ, the force acting perpendicular to ab is sinθ. Consequently, the torque Ta in part a is represented by the following formula:

Considering part C in the same way, the torque is doubled, generating a torque calculated by the following formula:

Given that the area of a rectangle is S = h*l, the following result can be derived by substituting it into the previously mentioned formula:

This formula not only applies to rectangles but also other common shapes like circles. This principle underlies the operation of electric motors.
How does a motor turn?
1) Motors rotate using magnets and magnetic force.
Around a permanent magnet with a rotating axis:
① Rotate the magnet (to generate a rotating magnetic field).
② This follows the principle that opposite poles (N and S) attract each other, while like poles repel each other.
③ The magnet with the rotating shaft will then rotate.
This is the basic principle of engine rotation.
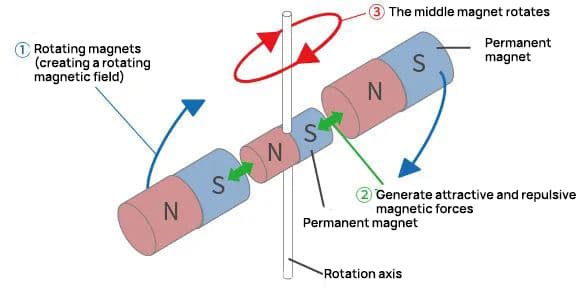
The current flowing through the conductor generates a rotating magnetic field around it, causing the magnet to rotate, which essentially represents the same state of motion.
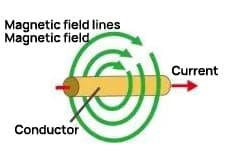
Furthermore, when a conductor is wound into a coil, magnetic forces are synthesized to form a large magnetic flux, creating a North pole and a South pole.
Furthermore, by inserting a ferrous core into the wound conductor, magnetic field lines can pass through more easily, thus generating a stronger magnetic force.
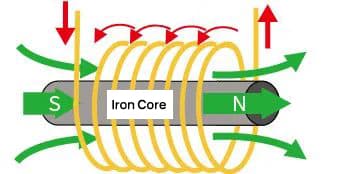
2) Operating Rotary Engines
In this context, we present a practical approach for rotary motors, demonstrating the use of three-phase alternating current and coils to generate a rotating magnetic field. (Three-phase alternating current refers to alternating signals phased at 120° intervals.)
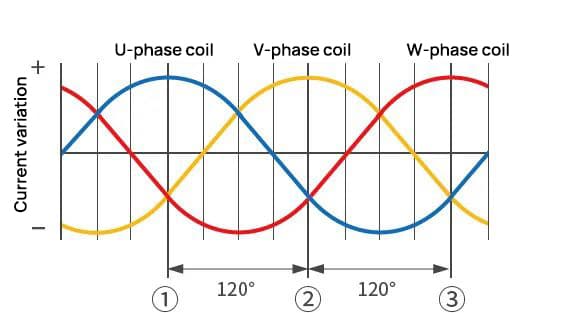
The magnetic field synthesized in said State ① corresponds to Figure ① below.
The magnetic field synthesized in state ② mentioned above aligns with Figure ② shown below.
The synthesized magnetic field associated with the aforementioned state ③ is depicted in Figure ③ below.
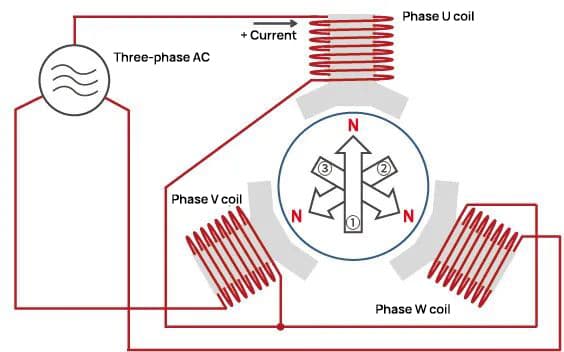
As stated above, the coil wound around the iron core is divided into three phases: U phase coil, V phase coil and W phase coil, arranged at 120° intervals. The coil with the highest voltage generates a north pole, while the one with the lowest voltage generates a south pole.
Each phase varies according to the sine wave, therefore, the polarity (north or south pole) and the magnetic field (magnetic force) generated by each coil will change.
At this point, if we consider only the coil that generates the north pole, the sequence of changes goes from the U phase coil to the V phase coil, then to the W phase coil, and finally back to the U phase coil. , thus producing a rotation.
Small Engine Structure
The figure below provides a general construction and comparison of three types of motors: stepper motors, brushed direct current (DC) motors, and brushless direct current (DC) motors.
The basic components of these motors mainly include coils, magnets, and rotors. Due to their diverse types, they are further classified into coil-fixed and magnet-fixed types.
stepper motor | Brushed DC Motor | Brushless DC Motor |
![]() |
![]() |
![]() |
The following is a structural description related to the example diagram. Please understand that this document presents the framework within a broad framework, as there may be other frameworks if broken down in more detail.
The stepper motor coils here are fixed on the outside, with the magnets rotating on the inside; The magnets of the brushed DC motor are fixed on the outside, with the coils rotating on the inside.
The supply of energy to the coils and the change in the direction of the current are carried out by brushes and commutators; In brushless motors, the coils are fixed on the outside, with the magnets rotating on the inside.
Due to variations in engine types, even with the same basic components, their structures may differ. Specific details will be explained in each section.
Brushed motor
Brushed Motor Structure
The image below shows the exterior of a brushed DC motor commonly used in models, as well as an exploded view of a typical two-pole (two magnets), three-slot (three coils) motor. Many may have experience taking apart motors and removing magnets.
In a brushed DC motor, the permanent magnets are stationary while the coils are allowed to rotate around the inner center. The stationary side is known as the “stator” and the rotating side is known as the “rotor”.
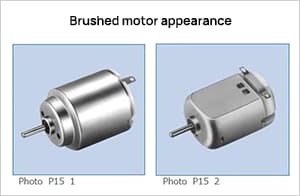
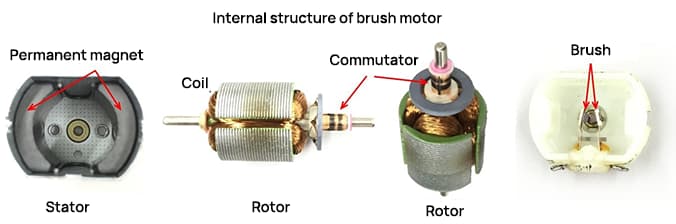
Here is a schematic diagram that illustrates structural concepts.
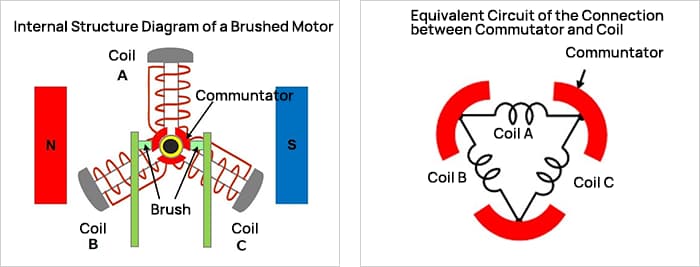
The central axis of rotation is surrounded by three commutators – curved metal plates used to switch electrical current. To avoid contact with each other, the switches are arranged 120° apart (360° ÷ 3 units). These switches rotate with the shaft.
Each commutator is connected to one end of a coil and the other, and together the three commutators and three coils form a complete circuit network (ring).
Two brushes are fixed at 0° and 180° to make contact with the commutators. An external DC power supply is connected to the brushes and current flows along the path from the brush to the commutator, from the commutator to the coil, and from the coil back to the brush.
This is the rotation principle of a brushed motor.
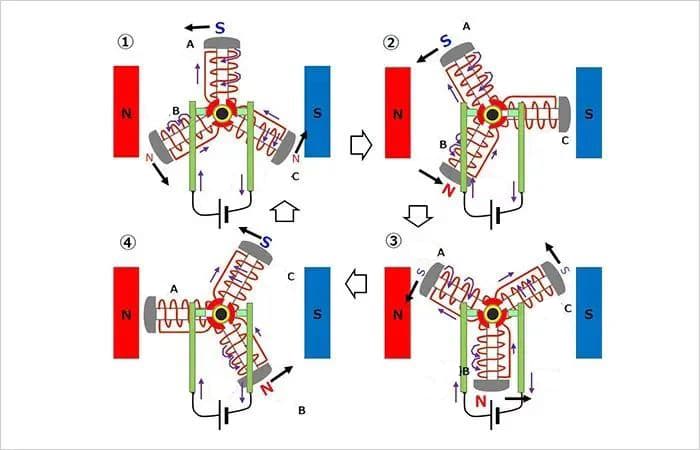
① Counterclockwise rotation from the initial state
Coil A is at the top, with the power supply connected to the brushes, designating the left side as (+) and the right as (-). A high current flows from the left brush through the commutator to coil A. This turns the top (outer) part of coil A into a south pole (S).
Because half of coil A's current flows from the left brush to coils B and C in the opposite direction to coil A, the outer sides of coils B and C become weak north poles (N), indicated by smaller letters in the diagram.
The magnetic fields generated in these coils, along with the repulsion and attraction of the magnets, provide a force that rotates the coils counterclockwise.
②Additional counterclockwise rotation
Then, assuming that coil A has rotated 30° counterclockwise, the right brush comes into contact with the two commutators.
The current in coil A continues to flow from the left brush through the right brush, and the outer side of the coil remains a south pole. The same current flows through coil B, turning its outer side into a stronger north pole. Coil C is short-circuited by the brushes at both ends, so no current flows and no magnetic field is generated.
Even in this state, a counterclockwise rotating force is applied. From ③ to ④, the upper coil continues to be moved to the left and the lower coil continues to be moved to the right, resulting in continuous counterclockwise rotation.
Each 30° rotation of the coil to states ③ and ④, when the coil is above the central horizontal axis, its outer side becomes a south pole; when it is below, it becomes a north pole and this movement repeats itself.
In other words, the top coil repeatedly experiences a force to the left, and the bottom coil a force to the right (both in a counterclockwise direction). This keeps the rotor spinning counterclockwise.
If the power supply is connected to the opposite left brush (-) and the right brush (+), a reverse magnetic field is generated in the coil, thus reversing the direction of the force applied to the coil, causing it to rotate clockwise.
Furthermore, when the power supply is turned off, the brushed motor rotor stops rotating due to the absence of the magnetic field that drove its rotation.
Three-phase full-wave brushless motor
Appearance and structure of three-phase full-wave brushless motor
The image below shows an example of the appearance and structure of the brushless motor.
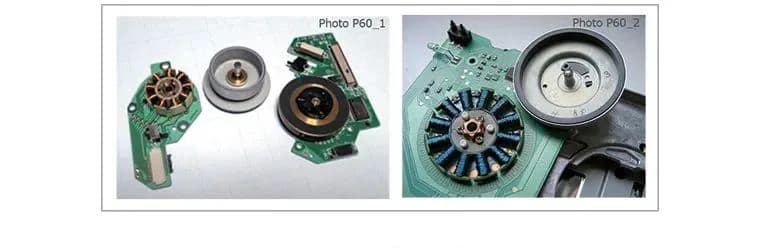
The left side shows an example of the main shaft motor used to spin the record in the record playback device, containing a total of nine coils, three phases times three.
On the right is an example of a main spindle motor from an FDD device, with twelve coils (three phases times four). The coils are mounted on a circuit board and wound on an iron core.
On the right side of the coil, the disc-shaped component is a permanent magnet rotor. The perimeter is a permanent magnet, the rotor shaft is inserted into the center of the coil and covers part of the coil, with the permanent magnet surrounding the periphery of the coil.
Internal structure diagram and equivalent circuit of three-phase full-wave brushless motor
Following are the simplified diagram of the internal structure and the equivalent circuit diagram of the coil connections for a three-phase full-wave brushless motor.
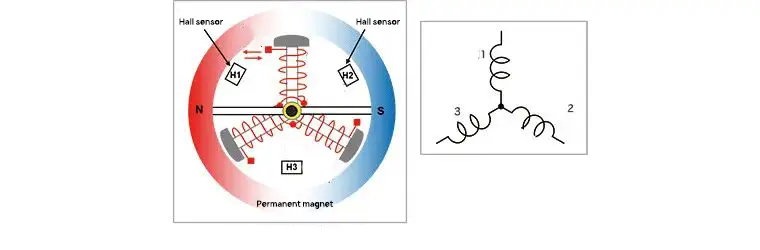
This schematic represents a simple structure of a 2-pole (2 magnets) and 3-slot (3 coils) motor. It resembles the structure of a brushed motor with equal number of poles and slots, except that the coil side is stationary and the magnets are rotating. Naturally, this design does not incorporate brushes.
In this configuration, the coils are connected in a Y formation. Semiconductor components supply current to the coils, controlling current input and output based on the position of the rotating magnets.
In this example, a Hall element is used to detect the position of the magnets. The Hall element is placed between the coils, where it detects the voltage generated by the intensity of the magnetic field and uses it to obtain position information.
In the previously provided image of the FDD spindle motor, a Hall element (located above the coils) can also be seen, which serves to detect the position between the coils.
The Hall element is a well-known magnetic sensor. It can convert the magnitude of a magnetic field into voltage, representing the direction of the field with positive or negative values. Below is a diagram illustrating the Hall effect.
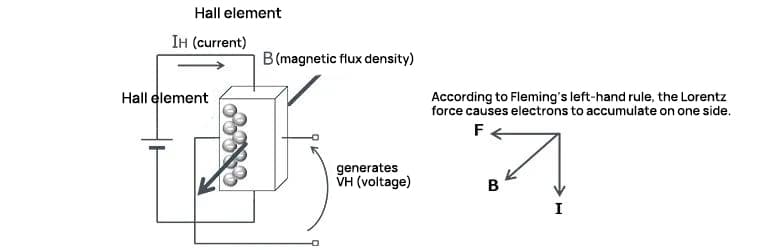
The Hall element uses the phenomenon that “when current IH flows through the semiconductor and magnetic flux B passes at right angles to the current, a voltage VH is generated in the direction perpendicular to the current and the magnetic field”.
This phenomenon, known as the “Hall Effect”, was discovered by the American physicist Edwin Herbert Hall. The generated voltage VH is represented by the following formula.
V H =(K H /d)・I H・B
Where KH is the Hall coefficient and d is the thickness of the flow penetration surface.
As the formula suggests, the greater the current, the greater the voltage. This feature is often used to detect the position of the rotor (magnet).
Rotation principle of three-phase full-wave brushless motor
The rotation principle of the brushless motor will be explained in steps ① to ⑥. To make it easier to understand, the permanent magnet has been simplified from a circle to a rectangle.
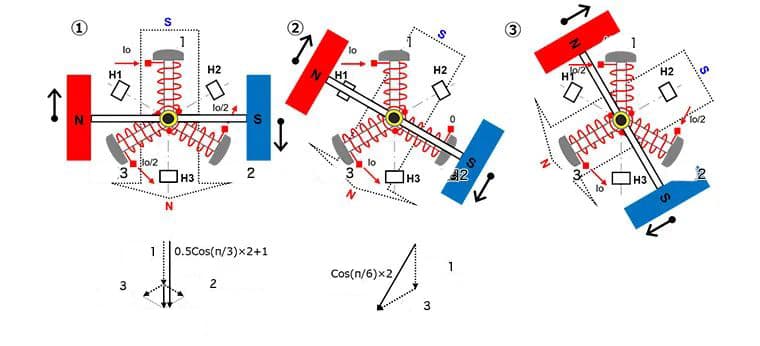
1) In a three-phase coil system, imagine coil 1 fixed at the 12 o'clock position on a clock, coil 2 at 4 o'clock, and coil 3 at 8 o'clock. Suppose a 2-pole permanent magnet with the North pole on the left and the South pole on the right, capable of rotation.
The current Io is introduced into coil 1, generating a South pole magnetic field on the outside of the coil. Half of this current, Io/2, flows out of coils 2 and 3, creating a North Pole magnetic field outside.
When the magnetic fields of coils 2 and 3 undergo vector synthesis, a descending North Pole magnetic field is generated. This field is half the size of the magnetic field produced when current Io passes through a coil and, when added to the field of coil 1, becomes 1.5 times larger. This creates a magnetic field synthesized at a 90° angle to the permanent magnet, thus generating maximum torque and prompting the permanent magnet to rotate clockwise.
As the current in coil 2 decreases and that in coil 3 increases based on the rotational position, the synthesized magnetic field also rotates clockwise, continuing the rotation of the permanent magnet.
2) With a rotation of 30°, current Io enters coil 1, setting the current in coil 2 to zero and causing current Io to flow out of coil 3.
The outside of coil 1 becomes the S pole, and the outside of coil 3 becomes the N pole. During vector combining, the magnetic field generated is √3 (approximately 1.72) times that of a single coil carrying current Io. This also results in a magnetic field compounded at a 90° angle to the field of the permanent magnet, rotating clockwise.
When the input current Io in coil 1 is reduced according to the rotational position, the input current in coil 2 starts to increase from zero and the output current in coil 3 increases to Io, the composite magnetic field also rotates in the direction clockwise, and the permanent magnet continues to rotate.
Assuming all phase currents are sinusoidal, the current here is Io×sin(π⁄3)=Io×√3⁄2. Through vector combination of the magnetic field, the total size of the magnetic field is 1.5 times the field produced by a single coil ((√3⁄2)2×2=1.5). When all phase currents are sinusoidal, regardless of the position of the permanent magnet, the size of the vector composite magnetic field is always 1.5 times that of a single coil, and the magnetic field forms a 90° angle with the field. permanent magnetic. magnetic.
3) After additional rotation of 30°, current Io/2 flows into coil 1, current Io/2 enters coil 2 and current Io flows out of coil 3.
The outside of coil 1 becomes the S pole, the outside of coil 2 also becomes the S pole, and the outside of coil 3 becomes the N pole. During vector combination, the magnetic field generated is 1.5 times the magnetic field produced when current Io passes through a single coil (same as in ①). A compound magnetic field is also formed here at an angle of 90° to the field of the permanent magnet, rotating clockwise.
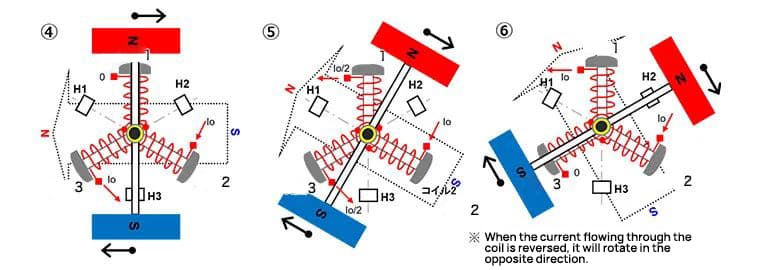
In steps 4 to 6, rotate in the same way as in steps 1 to 3.
In this way, by sequentially alternating the current flowing into the coil according to the position of the permanent magnet, the permanent magnet will rotate in a fixed direction. Likewise, if the current is reversed and the direction of the synthetic magnetic field is reversed, it will rotate counterclockwise.
The diagram below continuously displays the current for each coil in each of the steps 1 to 6 mentioned above. From the above introduction, the relationship between changes in current and rotation should be understood.
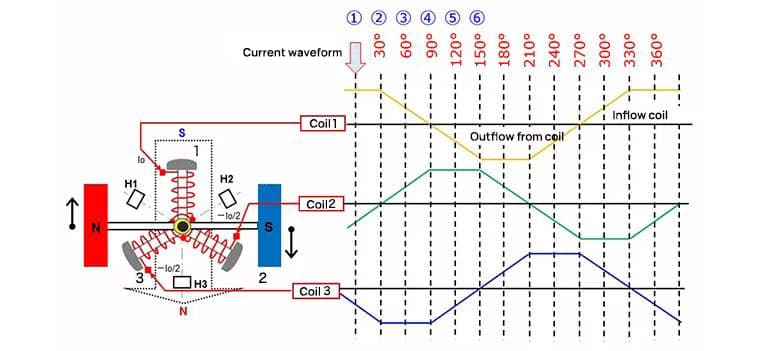
stepper motor
Stepper motor is a type of motor that can precisely synchronize pulse signals to control rotation angles and speed. It is also known as a “pulse engine”.
The stepper motor, which does not require a position sensor and can realize precise positioning through open-loop control, is widely used in equipment that requires precise localization.
Structure of a stepper motor (two-phase bipolar)
The images below, from left to right, show an example of the external appearance of a stepper motor, a simple diagram of its internal structure, and a conceptual schematic of its design.
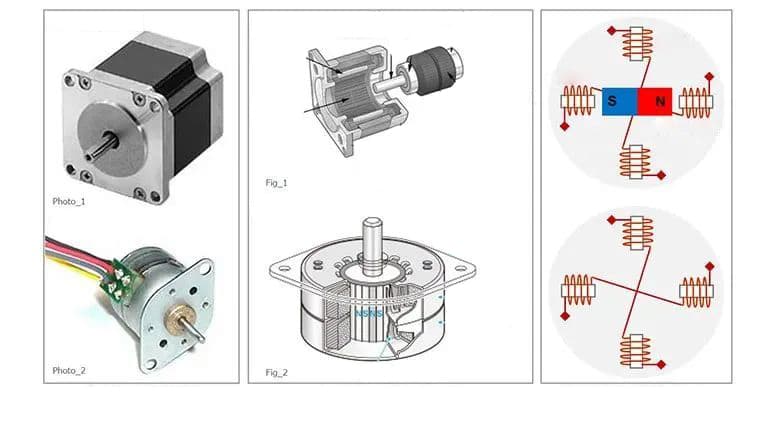
The external examples provided are HB (Hybrid) and PM (Permanent Magnet) stepper motors. The middle diagrams also depict the structures of HB and PM types.
Stepper motors are structured with fixed coils and a rotating magnet. The right-hand conceptual diagram of the internal structure of the stepper motor illustrates an example of a PM motor using two phases (two sets) of coils. In the basic structure examples of stepper motors, the coils are positioned externally and the permanent magnet is located internally. In addition to two-phase, there are also types with a greater number of phases, such as three-phase and five-phase.
Some stepper motors have different structures, but to explain their working principles, this article provides the basic structure of stepper motors. Through this article we intend to understand that stepper motors mainly adopt a structure with fixed coils and a rotating permanent magnet.
Basic Working Principle of Stepper Motors (Single-Phase Excitation)
The following diagram is used to explain the basic working principle of stepper motors. This is an example of single-phase excitation (one set of coils) of the two-phase bipolar coils mentioned above. The premise of the diagram is the change of state from ① to ④. The coils consist of Coil 1 and Coil 2. Furthermore, the current arrow represents the direction of current flow.
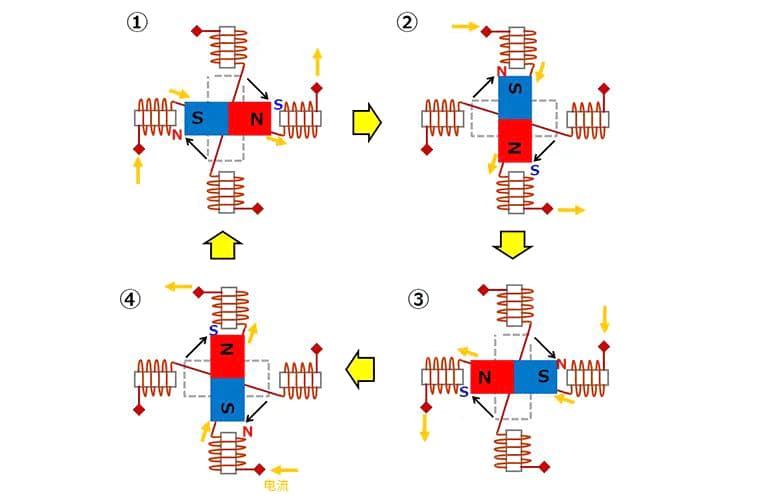
①Direct the current to enter the left side of coil 1 and exit the right side. Avoid any current flow through coil 2. As a result, the inner side of left coil 1 becomes N (north), while the inner side of right coil 1 becomes S (south). Consequently, the middle permanent magnet is attracted by the magnetic field of coil 1, adopting a state with S on the left and N on the right, and stops.
②Next, interrupt the current in coil 1, directing it to enter through the upper part of coil 2 and exit through the lower part. The inner side of the upper coil 2 then becomes N, and the inner side of the lower coil 2 becomes S. The permanent magnet is attracted by this magnetic field, turning 90 degrees clockwise and stopping.
③After that, interrupt the current in coil 2, directing it to enter from the right side of coil 1 and exit from the left side. The inner side of left coil 1 becomes S, and the inner side of right coil 1 becomes N. The permanent magnet is again attracted by this field, rotating another 90 degrees clockwise and stopping.
④Finally, interrupt the current in coil 1, directing it to enter through the lower part of coil 2 and exit through the upper part. The inner side of the upper coil 2 becomes S, while the inner side of the lower coil 2 becomes N. Once again, the permanent magnet is attracted by this magnetic field, turning 90 degrees clockwise and stopping.
By changing the current flow through the coils in the above sequence (① to ④) through an electronic circuit, the stepper motor can rotate. In this example, each switch action rotates the stepper motor 90 degrees.
Additionally, maintaining a continuous current flow through a specific coil allows the motor to remain in a stopped state while maintaining torque. As a side note, reversing the order of current flow through the coils can cause the stepper motor to rotate in the opposite direction.